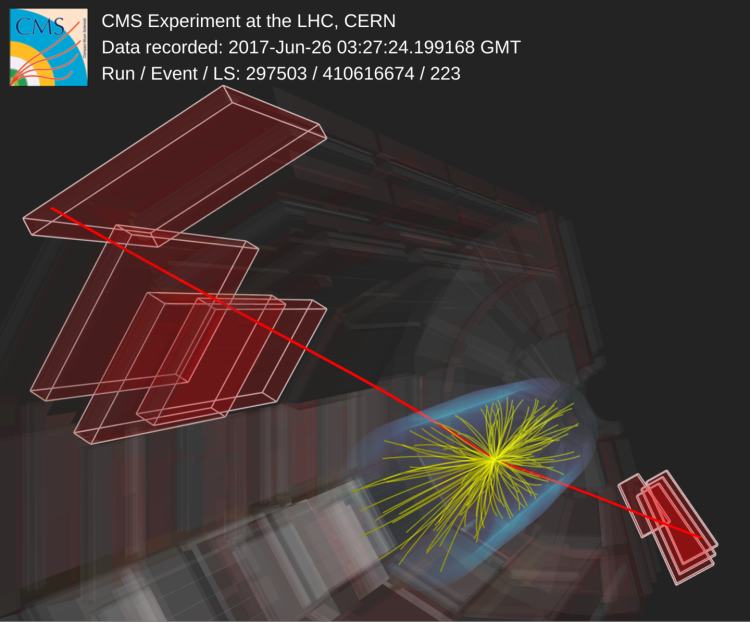
The more collisions the better, right? To search for new physics, the aim of the LHC experiments at CERN is to collect and investigate as many proton-proton collisions as possible to increase the chances that undiscovered particles are created and found. On the other hand, the effects of new physics might already be present in our current data and show up as small deviations from the known processes. To study this case, physicists perform measurements of the probability that a known process appears with the best possible precision. This probability is called the cross section (𝜎) and is measured in units of area. Because some processes are known to appear very rarely, i.e. have a small 𝜎, more collisions are needed for precise measurements of those as well. The value of 𝜎 is obtained by measuring the number of times a process occurs (N) within a sample of data of a given size, expressed by the so-called integrated luminosity (L). This means that accurate cross section measurements require not only a high number of collisions but also a precise knowledge of L.
At the LHC, bunches of trillions of protons cross every 25 nanoseconds, which is 40 million times per second. Typically, each time the bunches cross not only a single proton-proton collision happens, but multiple ones simultaneously, known as pileup. This increases the luminosity recorded per second, the so-called instantaneous luminosity. In 2017, the CMS experiment took data with an average pileup of around 30 simultaneous collisions, which means an average of 1.2 billion collisions per second. In future LHC runs, the pileup is expected to increase by a factor of 6 or 7. This confronts physicists with a problem: with higher pileup it becomes more difficult to precisely measure the instantaneous luminosity, i.e., to know how many collisions happened.
Figure 1: Visualization of a real CMS event containing two reconstructed muons (red lines), detected by the silicon tracker and muon detector systems (red boxes). This represents a clear signature of a Z boson. The yellow lines are reconstructed tracks, mainly coming from pileup interactions. You can zoom and rotate, also in this separate page.
Seeking a solution, physicists have developed a novel approach to calculate the luminosity based on a particle well understood from other precision measurements – what better food could a farmer eat than his own grown carrots? The Z boson is a particle that occurs in large quantities and decays right away. In about 3% of the cases, often enough for this method, it decays to two muons, the heavier siblings of electrons. An event display that depicts such decay is shown in Figure 1. The muon is the particle for which the “M” in “CMS” stands for: muons are the particles that can be identified the best. And the number of Z bosons can thus be extremely well measured.
However, the cross section of Z boson production is not known well enough to derive direct conclusions on the luminosity. Instead, we measure the ratio between the number of Z bosons from two data samples, which is proportional to the ratio between the two corresponding integrated luminosities. The luminosity in one data sample is therefore inferred from that of the other. One of the data samples has low pileup, so that its luminosity is measured more precisely using conventional methods.
Figure 2: The instantaneous luminosity measured using the Z boson method (black points) and a conventional measurement (red lines), as a function of time from the start of a LHC fill in 2017.
This method has been compared to conventional measurements and we see a good agreement, as illustrated in Figure 2. The study highlights that a better precision (i.e., a smaller uncertainty) can be achieved using Z bosons. We have also seen that the method agrees with conventional luminosity measurements even for the highest values of instantaneous luminosity recorded by CMS, as shown in Figure 3. The approach will therefore be suitable also in the future, when the experiments will experience unprecedented pileup levels.
Figure 3: The cross section of Z boson production, within the detector acceptance, as a function of the instantaneous luminosity, normalized by the average Z boson cross section. A non-negligible slope would indicate problems with either the conventional luminosity measurement or the Z boson measurement.
Read more about these results:
-
CMS Paper "Luminosity determination using Z boson production at the CMS experiment "
-
Display of collision events: CERN CDS
-
@CMSExperiment on social media: LinkedIn - facebook - twitter - instagram
- Do you like these briefings and want to get an email notification when there is a new one? Subscribe here