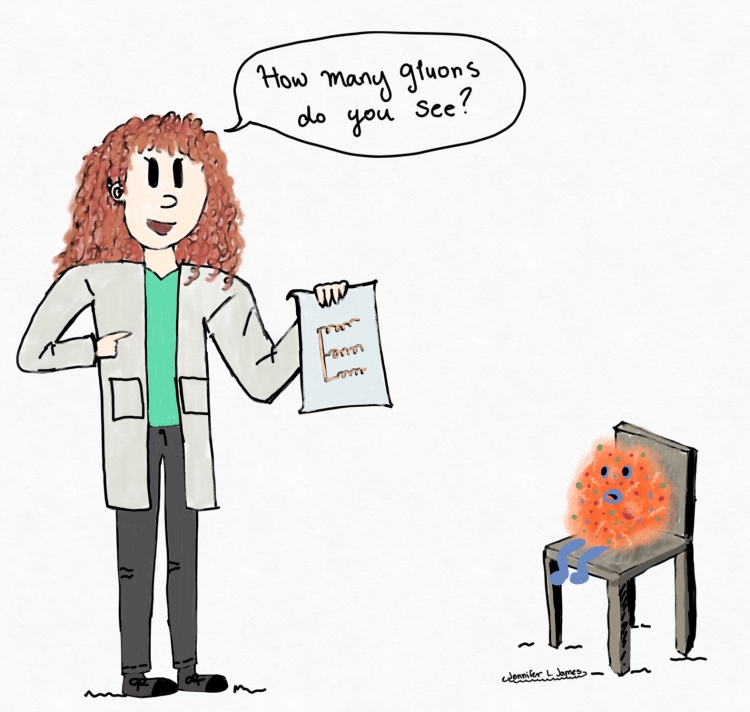
Image credit: Jennifer James (Vanderbilt)
Imagine going to an optician to check your vision. You are looking at a series of letters "E" in different orientations. When these letters get smaller and smaller, the horizontal lines in the "E" get closer and closer together, until eventually, you cannot tell which way the "E" is pointing. The letter is still there, but you would need better resolution in your eyes to resolve it. While getting a pair of glasses might do the trick for you, for quark-gluon plasma, a state of matter that was prevalent in the universe a fraction of a second after the Big Bang, glasses won't help. The quark-gluon plasma is actually predicted to suffer from a similar issue, which has a significant effect on its behavior.
While quark-gluon plasma existed in the early universe, in the modern day it can only be found in laboratories hosting giant particle colliders, like the Large Hadron Collider (LHC) at CERN. To create this elusive state of matter, we need to pack a huge amount of energy into a tiny volume. This is achieved by colliding heavy elements – in the case of the LHC, lead ions. The ions are accelerated close to the speed of light, and made to collide head-on. If the collision has enough energy, a droplet of quark-gluon plasma is created for a very short time, about 10-22 seconds (0.0000000000000000000001 seconds). The size of this droplet varies depending on the details of the collision, but it is of the order of a few femtometers in one dimension (10-15 m = 0.000000000000001 meters). This is enough for the scientists at the CMS experiment to study this matter, learning new insights about the basic laws that governed the universe in its first second of existence.
When the lead ions collide, a lot of particles are produced in addition to the quark-gluon plasma. Sometimes a highly energetic quark, a subatomic particle that protons and neutrons are made of, scatters from the collision. Quantum chromodynamics, the theory of the strong interaction, tells us that quarks can never exist alone. They must gather a posse around them. This leads to the emergence of jets, streams of particles that are concentrated in a small area, signaling that such a scattering has occurred. An example of how jets look in an experiment is shown in Figure 1.
Figure 1: Visualization of an event with two jets in heavy ion collisions. The yellow curves represent charged particles produced at the interaction point, while the green and blue boxes depict the energy of the particles. The two jets in opposite directions in the plane perpendicular to the beam are depicted by orange cones. The event display is interactive, you can zoom and rotate it, also on this separate page.
The presence of quark-gluon plasma affects this stream. It can make the quark emit gluons, carriers of the strong force, depleting the energy of the quark. It can also make the gluons further emit more gluons, but only if it can “see” them. This brings us back to the example at the optician. If the gluons are too close to the quark or other gluons, the plasma cannot resolve them, leading to fewer emissions and thus less depleted energy. The resolution angle, which determines which gluons are seen by the plasma, is a fundamental property of the plasma that is predicted to have a significant effect on the streams of particles that are seen in a detector. However, this angle has not yet been determined experimentally.
To see how badly quark-gluon plasma needs glasses, we take all possible pairs of particles within an observed stream, and measure the angles, Δr, between them, weighted by the product of the particles’ momentum components perpendicular to the axis of collision. This observable is called the energy-energy correlator. The comparison of energy-energy correlators measured from lead-lead collisions, where quark-gluon plasma is created, and proton-proton collisions, where it is not created, is shown in Figure 2. It can be seen that when the angle is small, both red and black distributions have quite similar shapes. However, something interesting happens at larger angles. The red distribution showing the angles with quark-gluon plasma starts to bend around Δr ~ 0.1. Even though it would be compelling to interpret this angle as the one where the quark-gluon plasma starts to see the emissions, there are also other possible explanations. For example, the jet might create a wake in the quark-gluon plasma. Imagine a duck swimming in a pond. When the duck treads the water, it leaves a triangular wake behind it in the water. If the same effect happens in quark-gluon plasma, we could also see this large angle enhancement.
Figure 2. Energy-energy correlator distributions measured from lead-lead collisions (red) and proton-proton collisions (black). Quark-gluon plasma is created only in lead-lead collisions.
These results provide an exciting new observable that has never before been measured in the presence of quark-gluon plasma. It shows striking behavior at large angles, which is a strong hint that quark-gluon plasma could indeed use prescription lenses.
Read more about these results:
-
CMS Paper (HIN-23-004): "Coming soon!"
-
Display of collision events: CERN CDS
-
@CMSExperiment on social media: LinkedIn - facebook - twitter - instagram
- Do you like these briefings and want to get an email notification when there is a new one? Subscribe here