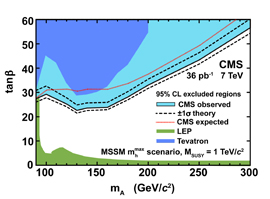
If you buy just about any LHC physicist a beer, and talk for a while, eventually you'll learn that he or she lives for the day when we make the next great discovery at this amazing machine. Many of them are placing their bets on finding the Higgs boson, the long-sought-after particle that gives mass to all the others. But what form will the Higgs boson take? It could be a single, simple particle, which is what the very successful Standard Model predicts. But the Higgs boson in the Standard Model has a problem — the parameters that determine its mass must be tuned incredibly finely for the model to work, akin to balancing a pencil on its sharpened point. If the Higgs mass parameters in the theory changes even a tiny bit, the calculated mass of the Higgs boson itself would be too high for us to observe.
And so physicists keep their options, and their eyes, open for other possibilities. One such possibility is that there is a symmetry in nature called supersymmetry, which would mean that for every particle we know about already, there is a super-partner which differs by half a unit of spin. In the simplest version of this model, the Higgs boson is not one but five particles: three neutral and two charged.
With the sample of physics data collected last year, CMS physicists have carried out one of the first searches for the neutral Higgs bosons in supersymmetry at the LHC. Ephemeral in nature, the Higgs boson strongly prefers to decay to the most massive particles that it can. The CMS team searched for cases where the Higgs bosons decays to pairs of (τ) tau leptons, the heaviest cousins of the electron. The nice thing experimentally is that there is a well-known particle, the Z boson, which also decays to tau pairs and serves as a “standard candle” to calibrate the Higgs boson search.
The tau leptons from the Higgs (or Z) are detected in CMS by the particles into which they decay. About 35% of the time, a τ will decay to a familiar electron or muon, plus two neutrinos that are not detected. CMS is particularly well-suited to triggering on collision events in which there are high-energy electrons or muons, and so we demand that at least one of the two τs decay this way.
The other 65% of τ decays give one or three charged pions, plus one or more neutral pions, and a neutrino, which escapes detection. The neutral pions give high-energy gamma rays that are well-measured in the electromagnetic calorimeter, and the charged pions leave tracks in the silicon tracking system. While these “hadronic” τ decays (i.e. decays into pions) are well measured, they are very similar to the “jets” of pions that come from quarks and gluons, which are much more numerous. The CMS team has developed powerful new algorithms to identify τ decays among the millions of jets.
The result of the search? No Higgs signal yet, but the search covered a large, previously unexplored region of supersymmetry parameter space, out to very high masses unattainable at Fermilab's Tevatron or earlier at LEP at CERN, as shown by the light-blue region on the graph below. Had the Higgs boson of suersymmetry been somewhere in that region, the CMS team would have found it. Fingers are crossed for this year's data — stay tuned!
Figure caption: The light-blue region shows the limits placed on the existence of the Higgs boson.
- Log in to post comments