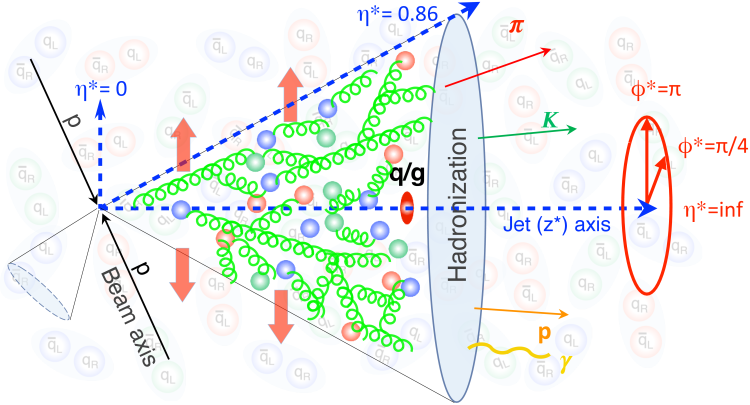
The universe is governed by four known fundamental forces: gravity, electromagnetism, the weak force, and the strong force. The strong force is responsible for dynamics on an extremely small scale, within and between the individual nucleons of atomic nuclei and between the constituents – quarks and gluons – that make up those nucleons. The strong force is described by a theory called Quantum Chromodynamics (QCD). One of the key details of this theory, known as “asymptotic freedom”, is responsible for both the subatomic scale of the strong force and the significant theoretical difficulties that the strong force has presented to physicists over the past 50 years.
Given the complexity of the strong force, experimental physicists have often led the research frontier and made discoveries that theorists are still trying to describe. This pattern is distinct from many other areas of physics, where experimentalists mostly search for and confirm, or exclude, theoretical predictions. One of the QCD areas where experimentalists have led progress is in the description of the collective behavior of systems with many bodies interacting via the strong force. An example of such a system is the quark-gluon plasma (QGP). A few microseconds after the Big Bang, the universe is supposed to have existed in such a state. The way the universe evolved in these brief moments and the structure that subsequently developed over billions of years is studied, in part, through experimental research on collective QCD effects. This briefing describes a recent exciting development in that research. To better understand the results, we begin with a series of analogies.
Imagine you are on a large university campus. You observe student movements in the middle of a busy exam period and find that the number of students entering the library in the morning is related to the number of students leaving in the evening. Perhaps this indicates some conserved quantity, like the number of students at the school. Each student in the library wants enough room to lay out their supplies and textbooks and get comfortable while studying. The library is nearly full and the students are evenly distributed across all the floors and halls of the library to ensure they have ample space. Recognizing and quantifying correlations like these can be useful for studying collective systems. By counting students “here” you can predict how many students are “there”, or by counting students “now” you can predict how many students you will get “later”. In this example, you may have insight into basic temporal and spatial correlations. However, you haven’t learned much about the social interactions of the students, a more interesting goal.
The university hosts an end-of-year concert that the whole school attends. The next day, everyone on campus is humming the same songs. Furthermore, you see many people wearing the same concert t-shirt. It turns out that a musician was distributing t-shirts to a few people, who in turn circulated them to others, until most people had one. Students who never met are now wearing the same t-shirts and humming the same songs. Correlated student behaviors have taught you a bit more about the interactions of people at the school. These types of correlations exist in systems with many moving parts and interactions. When heavy ions are accelerated towards each other at nearly the speed of light in the Large Hadron Collider, the resulting collisions produce highly interacting systems with many interacting quarks and gluons. Specific correlations within these systems have been used to study the formation and evolution of the QGP that existed in the early universe. In addition to correlations, physicists have also been successful in applying hydrodynamics, the theory of fluids, to study the evolution of these systems.
Let’s return to our university analogy. Another concert happens in the school’s stadium in the middle of summer, when the campus is nearly empty. It is poorly attended. After the concert, you see one person wearing a different concert t-shirt and humming new songs. Later, in a different part of campus you are shocked to see another person wearing the same second shirt and humming the same songs! The same types of correlations and student dynamics that existed for the huge concert appear to exist in the much more diluted and poorly attended concert. Somewhat surprisingly, the distribution of apparel and the campus-wide knowledge of the concert event occurred regardless of the concert size. This was the surprise found by CMS physicists in 2010. The much smaller and less dense system produced in proton-proton collisions showed the QGP-specific correlations previously only observed in collisions of much larger nuclei (gold, lead, etc.). This observation made it clear that the correlations were not unique to large fluid-like systems. Rather, they might be a general characteristic of any system whose fundamental properties are governed by the strong force.
Figure 1: Event display showing a proton-proton collision at 13 TeV in the CMS detector. Two high-energy jets are produced “back-to-back” and are easily identifiable with their energy deposits in the hadron (blue) and electromagnetic (green) calorimeters. One of the jets is composed of more than 100 charged particles (in blue) and represents the rare high-multiplicity jets that are of primary interest for this analysis. Try to zoom/rotate also as standalone here.
Physicists in the CMS Collaboration, in an effort led by Parker Gardner, Austin Baty, and Wei Li, of Rice University, have now taken one more step to understand what these correlations may offer, by studying the subset of rare proton-proton events that produce structures called “jets” (see an example in Fig. 1). These jets are collimated sprays of many particles that come from the evolution of single quarks or gluons. How a single particle transforms into a cluster of many individual particles (a process referred to as fragmentation or hadronization and illustrated in Fig. 2) is not yet perfectly understood. In the rarest cases, these processes can lead to more than 100 particles in the measured jet. In our analogy, we can think of a single concert goer from the summer event returning to the dormitory, which happens to be the largest dorm on campus, with 100 students. Only one of the 100 people participated in the event, but eventually the entire dorm is humming the music and demonstrating correlated behavior.
Figure 2: Illustration of a parton evolving to a shower that eventually exhibits collective expansion transverse to the jet axis. A jet cone (not to scale) and emerging final state particles are drawn in a coordinate system, denoted as the “jet basis”, where the z axis coincides with the jet direction. The redefined pseudorapidity (dotted blue lines) and azimuthal angle (solid red lines) are also represented.
The new study focused on these jets within the proton-proton collision system and found, for the first time, evidence suggesting that the rarest and most populated of these jets can develop correlations akin to those found in heavy-ion and high-multiplicity proton-proton collisions. The in-jet correlations span causally disconnected particles within the spray and provide very interesting information about the fragmentation and hadronization processes. Furthermore, these correlations are not predicted by several widely-used theoretical models and should spur significant advances in our understanding of the properties of the strong force. Figure 3 shows the two-particle angular correlation function, as a function of their separation in pseudorapidity, ∆η∗, and azimuthal angle, ∆φ∗, for two classes of particles: those from all jets (left panel) and those from the much smaller sample of jets with the highest in-jet track multiplicity (right panel, only 2 x 10^-5 of the jets contributing to the left panel). The “near-side” peak at (∆η∗, ∆φ∗) = (0, 0), truncated for visibility reasons, results from short-range correlations in the parton shower and hadronization, while the “far-side” ridge, at ∆φ∗ ≈ π, is related to back-to-back particle production and momentum conservation. These two features have also been found in lab-frame analyses of pp collisions and can be reproduced using simulations, indicating that the dynamics of bulk hadron production in the parton fragmentation process resembles those of a hadron-hadron collision process. Another interesting feature, observed in heavy-ion collisions and in high-multiplicity proton-proton collisions, is the “near-side ridge”, present at ∆φ∗ ≈ 0 over a wide range in ∆η∗. Such an enhancement is seemingly present in the right panel of Fig. 3 but is not seen in the left panel nor in the simulated event samples.
Figure 3: Two-particle angular correlation in the pseudorapidity and azimuthal angle 2D plane, for particles from all jets (left) and from jets with very high in-jet track multiplicity (right).
Figure 4: Single-particle elliptic anisotropy, v2∗, as a function of the in-jet track multiplicity. The two highest multiplicity data points clearly depart from the trend seen in the other data and in the model curves.
The 2D two-particle angular correlation distribution was integrated over the |∆η∗| > 2 “long range” to obtain the one-dimensional ∆φ∗ correlation, which was then turned into a Fourier harmonic spectrum. The resulting elliptic anisotropic harmonic, v2∗, decreases with the in-jet charged-particle multiplicity up to around 80, a trend well described by Monte Carlo event generators, and then shows an increasing trend for the rarest high-multiplicity jets, which is not reproduced by the models (see Fig. 4). This surprising observation offers new insights into the dynamics of the parton fragmentation processes.
Future studies will benefit from larger CMS event samples, collected with triggers specifically designed to select events with high-multiplicity jets. Additionally, other QGP signals, such as particle strangeness enhancement, will be examined in future analyses.
Read more about these results:
-
CMS Paper (HIN-21-013): "Observation of enhanced long-range elliptic anisotropies inside high-multiplicity jets in pp collisions at 13 TeV "
-
Display of collision events: CERN CDS
-
@CMSExperiment on social media: LinkedIn - facebook - twitter - instagram
- Do you like these briefings and want to get an email notification when there is a new one? Subscribe here