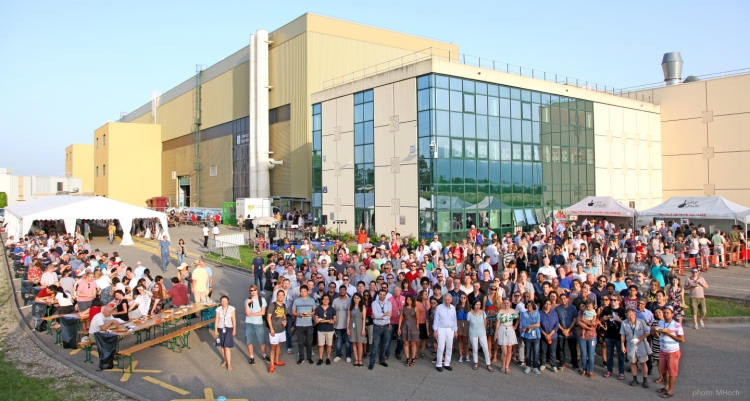
Not many people know that there is a boson-boson collider hiding in the proton-proton collisions of the Large Hadron Collider. The idea is that during rare occasions other particles such as the photon and gauge bosons W and Z are created by the protons in the LHC, and even more rarely two of those gauge bosons can collide.
An example of this is the neutral vector boson scattering (VBS) process. The VBS process can result in collisions producing jets in combination with Z bosons and photons, and this result looks for Zγ scattering, which is shown in Figure 1. In this process, two gauge bosons collide (in the figure, these are the two W bosons), to produce a Z boson and photon. From this, a treasure trove of information can be revealed about the subatomic world, such as the phenomenon of electroweak symmetry breaking. Electroweak symmetry breaking refers to how the electromagnetic and weak interaction forces once were unified but, at around a millionth of a millionth of a second after the Big Bang, this one force became two distinct forces.
Figure 1: An example Feynman diagram - a graphical representation of what happened in a collision - representing the vector boson scattering considered in this result: two quarks from the incoming protons (down and up) come from the left and produce two W bosons that interact in the center, and create the particles on the right. The W bosons form a Z boson and a photon on the right. On the right there are also still up and down quarks, these tend to point along the direction of the LHC beam.
In the Standard Model of particle physics, spontaneous symmetry breaking happens when the Higgs mechanism ends up providing some elementary particles with mass, such as the W and Z gauge bosons that transport the weak force. Meanwhile, other particles, such as the photon that carries the force of electromagnetism, remain massless.
Vector boson scattering is of great interest at the LHC experiments because it is sensitive to contributions that arise from the exchange of a Higgs boson, which in the Standard Model are not well defined at very high energies. One of the biggest questions to answer at the LHC after the Higgs discovery is to test the Standard Model predictions of the high-energy behavior of WW boson scattering.
It has only recently become possible to study VBS in detail thanks to the high energy and the high number of proton-proton collisions created by the LHC. Vector boson scattering has many striking characteristics including the presence of two widely-separated jets with a large invariant mass. Previously, in 2017, the CMS experiment observed the production of two W bosons of the same charge, with a significance of 5.7 standard deviations. Since then, there have been many more studies conducted by both the ATLAS and CMS collaborations. However, the Zγ vector boson scattering process is not yet discovered.
The CMS collaboration recently released a new measurement of the electroweak production of Zγ in association with two jets for the EPS-HEP conference. Data collected by the CMS detector in 2016 were analyzed, and the results were combined with previous results obtained using data collected in 2012. Combining the data helped to gain enough information to precisely measure vector boson scattering in the Zγ final state. The observed significance of the measurement is 4.7 standard deviations, while the expected significance is 5.5 standard deviations. Results close to 5.0 standard deviations indicate a one in a million chance of the result arising from a statistical fluctuation, and this threshold is commonly agreed to claim the discovery of new particles and collision mechanisms. This CMS study of VBS Zγ production is the first of its kind to have a high significance close to the discovery threshold.
This result is crucial in further understanding the nature of gauge interactions and how, and how often, gauge bosons can interact with themselves. The results can be used to reduce the possible range of different deviations from the couplings predicted by the standard model and provides the strongest limits to date to some of the “anomalous quartic gauge couplings” – deviations of the strength of the interaction between 4 gauge bosons. The distribution used to study the impact of anomalous quartic gauge couplings beyond the SM at high Zγ invariant mass region is shown in Figure 2.
Future LHC runs, and the data already recorded in 2017 and 2018, will allow CMS physicists to study the enigmatic vector boson scattering mechanism in much more detail.
Figure 2: This figure shows the invariant mass of the Zγ bosons selected to be consistent with VBS production. Anomalous quartic gauge couplings could modify the data (black points) according to the red line. The expectations from different processes are superimposed as filled, colored areas. The most significant deviations in the case of anomalous quartic gauge couplings are expected at high masses.
Read more about these results in the CMS Physics Analysis Summaries:
- Log in to post comments