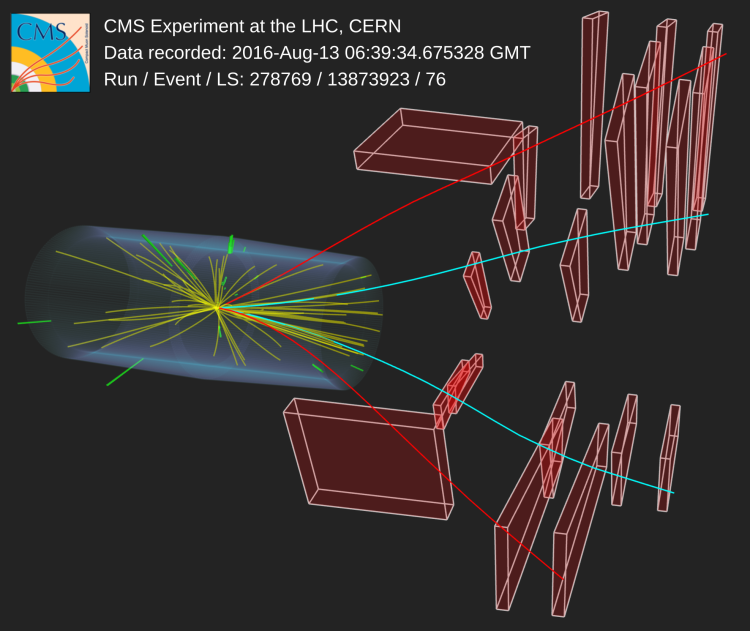
For over two millennia, people have wondered about the diversity of the material world, asking questions like “what are things made of?”. In the early decades of the 20th century we figured out that all things are composed of electrons, protons, and neutrons. Then, in the 1960s, it was discovered that protons and neutrons, which make up the atomic nuclei, are not elementary particles; they are composed of three quarks bound together by gluons, the agents of the strong force. Today, we know that quarks come in six "flavors": up (u), down (d), charm (c), strange (s), top (t), and bottom (b). Top quarks are too heavy to exist in a stable form: they decay immediately after being produced. The other quarks can bind together as quark-antiquark pairs (called mesons) or as quark triplets (called baryons); all particles composed of quarks are jointly known as “hadrons”. The protons and neutrons, for example, are uud and udd triplets, respectively; they are very well known because they are at the basis of all the matter around us. The J/ψ, composed of a charm quark and a charm antiquark (a “c-cbar”), is one of the most famous mesons: its discovery (by two independent experiments and simultaneously announced on November 11, 1974) proved the existence of the c quark and opened the door for a series of discoveries that placed the standard model of particle physics on solid grounds.
In 2003, the Belle experiment at KEK, in Japan, discovered a particle that did not seem to qualify as a standard meson or baryon: the X(3872). A possible interpretation is that it is a bound state of two quark-antiquark pairs (a tetraquark), one of them being a c-cbar pair. Quark systems composed of four or five quarks and/or antiquarks are called “exotic states” and present a new window to study how the quarks bind together. Many such states have been found by now, triggering the development of several theoretical models, undergoing heated scrutiny: the nature of these states remains unclear.
Figure 1: The measured double-J/ψ mass distribution and the results of fits with three signal peaks superimposed on a continuum background, before (left) and after (right) including the possibility of interference among the three states.
Inspired by indications seen in the relatively small 2011–2012 data samples, the CMS collaboration performed a search for such exotic states using events with 4 muons collected in 2016–2018. The strategy and analysis procedures were fixed before looking at the data samples (a method known as “blind analysis”). The search revealed candidates for three exotic states in events where they decay to two J/ψ mesons, which decay themselves to muon pairs. These states are seen in Fig. 1 as peaks labeled X(6600), X(6900), and X(7300). An example of a X(6600) candidate is shown in Fig. 2. The X(6900) was previously seen by the LHCb, CMS and ATLAS experiments. Both LHCb and ATLAS also reported a broad enhancement in the 4-muon mass spectrum close to threshold. Of the two new structures seen now by CMS in the J/psi J/psi mass spectrum, only the one at 6600 MeV has a significance that exceeds the famous 5 sigma threshold.
Figure 2: Event display showing a X(6600) state decaying to a pair of J/ψ mesons, followed by the decays of each J/ψ to two muons. The mass of the muon-pair represented by the red lines (blue lines) is 3.12 GeV (3.05 GeV) and the mass of the double-J/psi candidate is 6.52 GeV.You can view (zoom/rotate) the interactive event display on this separate page.
A tempting interpretation of these resonances is that they are all-charm tetraquark states (two c-cbar pairs). The production of a four-charm bound state is expected to be very rare: charm quarks are heavier than the quarks most abundantly produced at the LHC (u, d, and s). Producing a c-cbar pair costs energy (converted into the charm-quark masses by E = mc2). Producing two pairs at once, close enough and with the right characteristics to form a bound state, is much less probable. It is therefore quite exceptional that we have found three such states, a whole “family”. Having experimental evidence for the existence of three states is very exciting because the theoretical models provide much more precise calculations of the differences between the masses than of the mass values themselves. Besides, charm quarks are sufficiently heavy to ensure the reliability of a precise theory prediction, so that this new measurement is expected to provide a deeper understanding of hadron formation.
Figure 1-left presents the measured double-J/ψ mass distribution and the result of a fit with a model that only includes three peaks and a smooth continuum background; the dips between the three peaks are not well reproduced. As shown in Fig. 1-right, a better description of the data is obtained if the model includes a quantum effect, called “interference”, that enhances or reduces the signal strength at different mass values. One of the conditions to have interference is that the states must have identical “spin-parity” quantum numbers, so that the measurement might indicate that we are, indeed, seeing a family of states.
Besides the tetraquark interpretation, which is the simplest and most popular, other models are under consideration. Further advances in this physics area require more detailed measurements (spin-parity, production rates, etc.), under way using the proton-proton data currently being collected by CMS. Equally exciting would be to find exotic states composed of bottom quarks, as they would provide even more precise data-theory comparisons, given their higher masses.
Read more about these results:
-
CMS Physics Analysis Summary "Observation of new structures in the J/J/ mass spectrum in pp collisions at 13.6 TeV"
-
@CMSExperiment on social media: facebook - twitter - instagram
- Do you like these briefings and want to get an email notification when there is a new one? Subscribe here