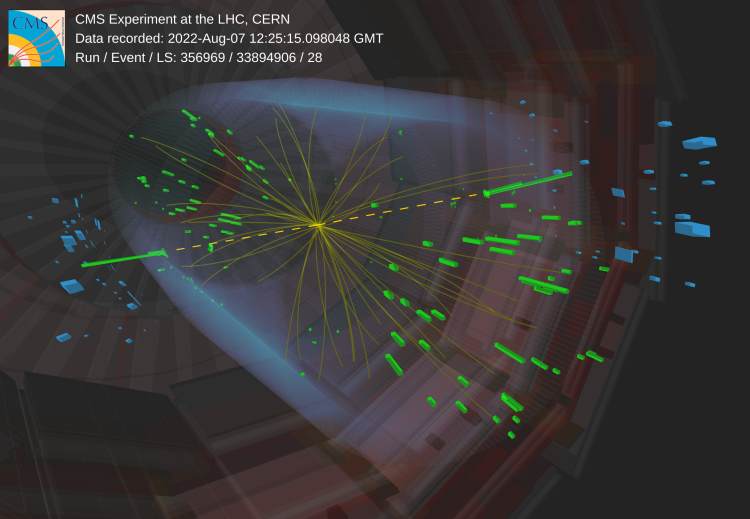
In 2012, the discovery of the Higgs boson marked a milestone in particle physics. It validated the standard model (SM) and unlocked new avenues of exploration. In 2022, the Higgs boson turned ten and the CMS experiment now presents a new measurement of Higgs boson production with data collected at the unprecedented centre-of-mass energy of 13.6 TeV.
The production of a Higgs boson in proton-proton interactions is a rare occurrence: There is a chance of less than one in a billion that the constituents of the colliding protons form the precious boson. To collect enough Higgs boson events, the LHC smashes bunches of up to 1012 protons into each other 40 million times per second, creating a very messy experimental environment with large amounts of signals from all sorts of particles. To make matters worse, Higgs bosons decay almost instantly after being produced. Thus, their presence can only be inferred by carefully measuring the decay products. In this measurement, physicists from the CMS experiment targeted the decay of the Higgs boson into the particles that make up light, photons. On the one hand, the signature of two energetic, isolated clusters of electromagnetic energy is striking, as can be seen in Figure 1. On the other hand, the LHC produces look-alikes of such diphoton decays in great quantities. To identify the needle in the haystack, physicists use sophisticated data analysis methods and machine learning techniques to reject background events and isolate the most wanted particle of the standard model.
Figure 1: Event recorded with the CMS detector in 2022 at a proton-proton centre-of-mass energy of 13.6 TeV. It shows characteristics expected from the decay of the SM Higgs boson to a pair of photons (dashed yellow lines and green towers). The event could also be due to one of the known background processes. It can also be viewed on a full, interactive page here.
Not every background event consists of genuine photons, though. Bundles of hadrons, called jets, can look like an energetic single photon. The CMS experiment utilises a machine learning technique called “boosted decision trees” to filter out these imposters and identify genuine photons. However, imperfections in the simulation of this algorithm can lead to large uncertainties in the measurement. The analysers have developed a new deep learning method to correct the estimation of the boosted decision trees based on the properties of electrons, the charged friend of the photon. “With this new technique, we can reduce the uncertainties of the measurement and improve our confidence in the simulation events of CMS”, remarked Caio Daumann, a PhD student at RWTH Aachen University, who devised this method. The excellent agreement of the corrected simulation with the data is shown in Figure 2.
Figure 2: Comparison between the observed data (black dots) and the uncorrected (blue) and corrected (green) score of the photon identification boosted decision tree in electron events. As shown in the lower panel, the agreement after correcting the simulation is excellent.
The search for the needle is performed by investigating the reconstructed mass of the system formed by the photon pair in each event. Even though the Higgs boson decays instantly, its echo can be felt in the configuration of its decay products: it leaves a fingerprint of a peak at its mass of about 125 GeV that can be reconstructed from the two photons (Figure 3). This feature is not present in background events, allowing the analysers to count the total number of produced Higgs bosons based on the shape of the observed mass distribution. In addition, for the first time, CMS data at an energy of 13.6 TeV were used to measure how the production cross section (the probability of a Higgs boson being produced) depends on the momentum and angle of the Higgs boson, as well as the number of jets. “The measured cross sections are in good agreement with the theoretical predictions. The Higgs boson has passed this first initial test at this record centre-of-mass energy”, comment Nico Härringer, PhD student at ETH Zürich, and Chengyang Pan, PhD student at Peking University, who played important roles in the statistical analysis of the data.
Figure 3: Reconstructed diphoton mass distribution in the measurement of the inclusive cross section. The signal+background fit reveals a tiny bump over the background-only assumption (upper panel), which is amplified by subtracting the background component (lower panel).
Overall, the re-observation of the Higgs boson in such a rare decay channel with 13.6 TeV data demonstrates the high quality of the collected data and the readiness of CMS to continue its rich physics programme. “This analysis leverages advanced deep learning techniques and modern analysis workflows. With this, we are already setting the stage for future measurements with much larger datasets that will be collected in the next fifteen years”, summarises Jan Lukas Späh, a PhD student at RWTH Aachen University, who performed the inclusive cross section measurement and coordinated the analysis team. Stay tuned for more studies of the Higgs boson with photons to come!
Written by: Jan Lukas Spah, for the CMS Collaboration
Edited by: Muhammad Ansar Iqbal and Andrés G. Delannoy
Read more about these results:
-
CMS Physics Analysis Summary (HIG-23-014): "Measurements of inclusive and differential Higgs boson production cross sections at 13.6 TeV in the H→𝛾𝛾 decay channel"
-
Display of collision events: CERN CDS , Full interactive event display here
-
@CMSExperiment on social media: Bluesky - Facebook - Instagram - LinkedIn - TikTok - Twitter/X - YouTube