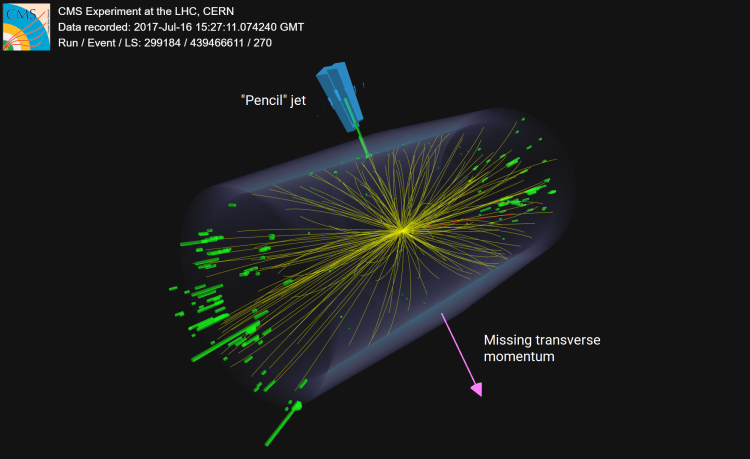
The CMS experiment conducts the first search for dark matter particles produced in association with an energetic narrow jet—the pencil jet.
Dark matter remains one of physics’ greatest mysteries. Despite making up about 27% of the universe’s energy content, its true nature is unknown. Astonishingly, all ordinary matter—which includes stars, planets, our phones, the wires transferring data, the waves carrying WiFi, you and me—accounts for just 5% of the total energy content of our universe. If our known world is so diverse, the dark sector, which outweighs it 20-to-1, could be just as rich. At CERN’s CMS experiment, scientists are searching for dark matter particles, aiming to reveal their interactions and revolutionize our understanding of our universe.
But if dark matter is so abundant, why haven’t we detected it? As the name suggests, dark matter does not interact with light (electromagnetism) with the same strength as ordinary matter (the behaviour of which is explained by the Standard Model) and, so far, is only known to interact with known particles through gravity, the weakest of the four known fundamental forces. At CMS, scientists use momentum conservation to infer the presence of dark matter: missing momentum in particle collisions (after accounting for detector mismeasurements) could signal an invisible particle, possibly dark matter, slipping away undetected.
In addition to particles that make up dark matter, there could be as yet undetected particles that mediate interactions between the dark particles and the matter particles. These are creatively called, you guessed it, mediators. Such mediators are bosons, implying that they carry integer spin quantum numbers as opposed to fermions (e.g. electrons) which have half-integer spins. One such mediator is the hypothesized Z' boson, which is electrically neutral and has spin quantum number of 1.
Above: A schematic (Feynman) diagram of the production of dark matter particles, one of which radiates a light Z' boson that creates a narrow (pencil) jet consisting of standard model particles.
Typical CMS searches focus on heavy Z' particles in the hundreds of GeV to TeV range, but a lighter Z' boson could also exist in the dark sector. It is typically a lot more challenging to look for such light particles due to the overwhelming background from hadronic resonances and quantum chromodynamics (QCD) processes—related to the strong nuclear force—which are poorly modeled at low energies. This is where techniques like data augmentation and machine learning can be utilized, enhancing sensitivity to Z' decays while suppressing known background processes.
The Z' boson mass that we are looking for in this search is around 1 GeV, and because of the low mass and high boost (momentum) of the Z' boson, it can only decay to light quarks (u, d, s), which then hadronize to form a jet (a spray of particles) with a lower number of constituents than usual. We then look for dark matter recoiling against such a narrow jet (called a pencil jet). This is the first search at the LHC for this signal. Various selections are applied to reduce the background processes while retaining the signal process and a combination of neural networks and boosted decision trees are used to further extract the signal. Multiprocessing techniques are used to speed up the processing time of the events.
“The main challenge in this analysis of real-world data was that the physically motivated input features aren’t typically well modelled in our simulations and so we had to take steps to ensure model robustness. We showed that using machine learning can help us achieve up to 10 times more sensitivity to these rare signal processes compared to traditional strategies” says Abhishikth Mallampalli, a graduate student at the University of Wisconsin-Madison, leading this search. Statistical hypothesis testing is used to determine whether the observed data agrees with the standard model prediction or suggests the presence of a dark matter signal.
We see that the data agrees well with the standard model expectation across the three years of proton-proton collision data analyzed. While this means that such a Z' boson with the probed light masses might not exist in our universe at the 95% confidence level, null results in such searches for dark matter not only solidify the standard model but also serve as guidance to theorists in building new physics models for dark matter, and help experimentalists to identify the direction for future searches.