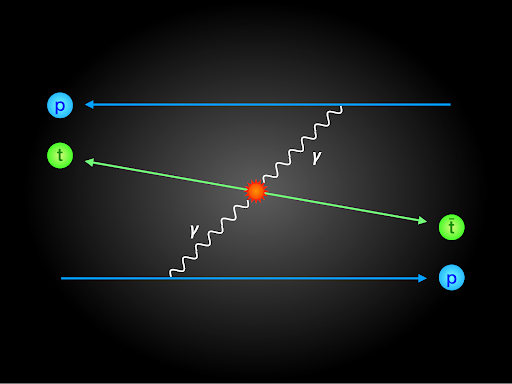
Ask someone what experiments at the CERN-LHC do, they will tell you: ‘You accelerate two protons to very high energy, smash them together and look in great detail at all particles created from the disintegration of the protons.’ etcetera. Well… yes… mostly. While this kind of event constitutes the main focus of the experimental program at the LHC, not all proton-proton interactions proceed in the same catastrophic way. It often happens that one or both of the interacting protons lose only a small part of their energy in the collision and continue their run along the LHC beam pipe. Since the LHC magnets are tuned to guide full-speed protons, the slightly slower protons would progressively drift away from their original path. Here is where the CMS-TOTEM Precision Proton Spectrometer (CT-PPS) experiment comes into play. An array of tracking and timing devices are suitably installed along the beam line, at about 200 m from the CMS interaction point, on both sides. These detectors are parked in their "garage" positions during the beam acceleration phase and moved towards the beam within a mere 2 to 3 mm, when the LHC machine is in the stable operation mode. This way, the tracks of displaced protons that have lost from, say, about 2% to 20% of their initial energy can be reconstructed. Figure 1 displays the position of one arm of the CT-PPS detector in the LHC tunnel, with two tracking stations indicated by the light blue arrows.
Figure 1: An end on view of the LHC tunnel in the region where the CT-PPS detectors are installed, for one of the two arms of the spectrometer. The CMS interaction point is about 200 m behind. The two light blue arrows show where the two tracking stations used for the measurement described here are located.
That’s all very well; but what happens to the energy “lost” by the protons? It goes into the creation of more particles! Since the initial energy of each proton in the LHC is so amazingly high (on particle scale), equivalent to about 6500 times the mass of the proton (about 1 GeV in high energy physics parlour) itself, even a relatively small loss can result in the creation of many different sets of final particles. Take the heaviest particle known to date, the top quark, which has a mass corresponding to about 2.5% of the initial energy of each proton, ie, about 173 GeV. This means that, in principle, occasionally there will be sufficient energy accumulation to produce one or two top quarks (remember E =mc2), of course the probability being driven by the quantum laws. A pair of lighter particle-antiparticle would require less energy to be produced and such an occurrence will be more frequent. In the “photon-photon fusion” process one proton from each of the incoming beams lose some amount of energy by emission of a photon; these two photons then convert their total energy into the creation of a top quark and anti-quark (tt) pair. The protons remain intact and nothing else other than this tt pair is produced in this exclusive production process.
The standard model predicts an exceedingly low probability for this process to occur: only about once in every four hundred thousand billion proton-proton interactions on average. So, its observation in the data sample collected so far, corresponding to about one thousand billion proton-proton interactions, would require a substantially higher rate for the process. Hence it would be be a striking manifestation of what physicists conventionally call “New Physics”, the physics beyond standard model.
Scientists of the CMS and TOTEM collaborations have looked for evidence of this process in the data collected at the LHC in 2017. To this end, they have selected tt candidates from the product of their decays reconstructed in the central CMS detector, and a proton on both sides of the interaction point from the tracks reconstructed by the CT-PPS detector. The top quark being an unstable particle, it decays mostly via a W boson and b quark. The W bosons are also transients and they decay, in turn, either leptonically (electron/muon/tau and a corresponding neutrino) or, hadronically, to a pair of quarks which produce jets or sprays of particles in the detector.
Figure 2: A computer reconstruction of a collision recorded by CMS which passes the signal selection criteria. The two blue lines with arrows are the protons that escaped intact from the interaction point and whose tracks have been reconstructed by the CT-PPS detectors, about 200 m away. The remaining objects are those reconstructed by the central CMS detectors, and constitute the decay products of a candidate tt pair. The two jets of particles originating from the b quark produced in the decays of the tops are rendered with the orange cones. One of the two W bosons in this event is reconstructed from its decay in two lighter quarks (up, down, strange, or charm), generating the jets represented by the yellow cones; the other is reconstructed from its decay to a muon, indicated by the red line, and a neutrino (which escapes undetected but causes an unbalance in the visible energy). You can view the 3-dimensional display interactively here.
Figure 2 presents an image of one of the collisions recorded by CMS experiment and selected for this study. The two blue lines with arrows are the protons that escaped intact from the interaction point and whose tracks have been reconstructed by the CT-PPS detectors, about 200 m away. The remaining objects are those reconstructed by the central CMS detectors, and constitute the decay products of a candidate tt pair. Top quarks decay in almost 100% of the times to a bottom (b) quark and a W boson. The jets originating from b quarks are rendered with the orange cones. One of the two W bosons in this event is reconstructed from its decay in two lighter quarks (up, down, strange, or charm), generating the jets represented by the yellow cones; the other is reconstructed from its decay to a muon, indicated by the red line, and a neutrino, which escapes undetected but causes an imbalance in the visible energy. The red, light blue and green blocks represent signals recorded by the subsystems of the CMS detector. You can also interact with the image separately here .
Nothing else is expected to show up in this interaction. However, protons in the LHC beams are so densely packed in “bunches” that each time two bunches collide several interactions happen almost simultaneously, thus making the quest significantly harder. Specific properties of the events sought can be measured and combined judiciously to help discriminate against those that just look alike (“the background”); yet the size of this background contribution is such that it would take a rather big signal to stand out of it. At the end of their analysis, the scientists could not find any evidence of the p p → p tt p process, but were able to exclude the hypothesis that it occurs much more often than what the SM predicts: the rate at which it happens cannot be larger than about 2000 times the standard model value. That still leaves some leeway for physics beyond standard model to show up, relatively meekly though, you never know. More importantly, this analysis demonstrated, for the first time, the feasibility of such a measurement, and thus has set the stage for more ambitious efforts in the years to come, with larger data samples and upgraded detectors.
Read more about these results: