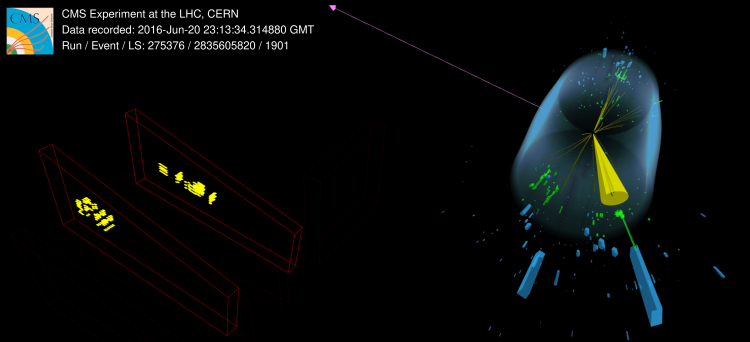
Most searches for new physics beyond the standard model (SM) focus on particles that decay almost instantly after being produced. However, many theories predict particles that travel a long distance, even up to meters, before decaying. Observing these "long-lived particles" (LLPs) at the LHC requires a creative use of the detector.
This search for physics beyond the SM targets a twin version of the Higgs boson that allows communication between the SM particles, that we can call “visible sector”, and a new, invisible “hidden sector”. The two sectors can talk to each other via SM Higgs boson decays into neutral long-lived particles. The search also looks for “dark QCD” models, a mirror version of QCD in which the SM Higgs boson decays to “dark quarks”, that hadronize into long-lived “dark mesons”. These LLPs may have decay lengths up to several meters, hence traveling through the inner detector layers without leaving any trace. They decay into SM particles only when they reach the outermost part of the CMS detector, the muon system. The decay products of the LLPs then interact with the shielding material, producing a shower of secondary particles that ionize the gas in the muon detectors, thus generating a large multiplicity of hits that cluster together and are identified as a muon detector shower. Figure 1 shows a collision event compatible with the signature of a Higgs boson decaying to LLPs.
Figure 1: A proton-proton collision at a center-of-mass energy of 13 TeV, recorded by the CMS experiment, compatible with the production of a Higgs boson decaying to long-lived particles that shower (orange lines) in the cathode strip chambers of the muon detectors system (red boxes). The event also contains a jet (yellow cone) and missing transverse energy (pink arrow). You can also try to rotate and zoom on this separate page.
To identify muon detector showers requires a non-standard reconstruction algorithm, since no “standard” particle creates this kind of signature. On one hand, this quirky behavior makes a LLP decay clearly distinguishable from the background processes, given the very low likelihood of accidentally having a muon shower induced by SM particles. On the other hand, it is very challenging to treat the muon detectors system, designed to identify the trajectory of muons, as a calorimeter collecting the hits generated in the electromagnetic or hadronic LLP decays.
In this search we combine, for the first time, the muon showers reconstructed in the forward muon system with those reconstructed in the barrel muon system. We split the events in three categories: events with only one shower in the barrel, only one shower in the forward region, or two showers (anywhere). Each category is studied independently. The analysis strategy and the extended geometrical coverage allowed us to achieve an unprecedented sensitivity to the twin Higgs and dark showers models.
In all three categories, the “size” of each cluster, namely the number of hits it contains, is the most discriminating variable. The signal showers have a larger multiplicity of hits than those accidentally generated by background processes. Backgrounds consist mostly of jets that are not fully contained in the calorimeters and reach the first chambers of the muon system, energetic muons that radiate photons in the muon chambers, and hadrons due to secondary interactions produced in the proton collisions. As an example, we show the number of hits in each cluster for the forward and muon barrel detectors in Fig. 2.
![]() |
![]() |
Figure 2: Distributions of the number of hits per cluster observed in data (black markers) and expected for the searched signal (colored histograms), when the cluster is reconstructed in the forward (left) and barrel (right) muon detectors.
The background contribution is estimated directly from data. No significant deviation from the expected SM sources has been observed and we could set the most stringent upper limits to date on the Higgs boson decay to a pair of LLPs with masses below 10 GeV. We also set the first LHC upper limits on the branching fractions of the Higgs boson decay to dark quarks, down to 10−3, in the framework of dark shower models.
Our quest for LLPs is not over yet! Several developments have been implemented for the ongoing LHC Run 3. First of all, we have introduced the muon shower object at trigger level. With the new triggers we increase the signal detection efficiency by at least an order of magnitude. We are currently working on analyzing the data collected in 2022 and 2023; stay tuned!
Read more about these results:
-
CMS Physics Analysis Summary "Search for long-lived particles decaying in the CMS muon detectors in proton-proton collisions at 13 TeV"
-
@CMSExperiment on social media: LinkedIn - facebook - twitter - instagram
- Do you like these briefings and want to get an email notification when there is a new one? Subscribe here