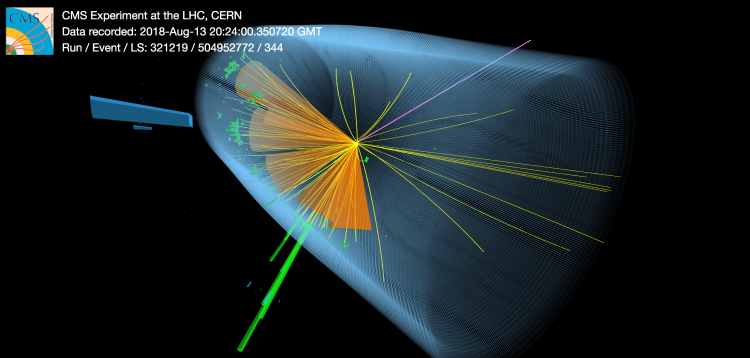
The CMS Collaboration has recently presented an updated search for new invisible particles. The technique capitalizes on the imbalance among the visible particles in a collision that such an object would induce. If dark matter is made of fundamental particles, these could reveal themselves in just this way.
The standard model is used to arrange elementary particles into groups that describe their interactions and has predicted the existence of some particles, such as the Higgs boson, years before their discovery. The standard model assigns the known particles into matter particles (fermions) and force-mediating particles (bosons). This simple classification assigns particles to big groups according to the way they behave. For example, an electron is a fermion, which gives rise to the electron cloud shapes around the nucleus of an atom. When electromagnetic radiation, including light, exhibits its particle character as a photon, that particle is a boson.
The standard model has several weaknesses; for example, the particle nature of Dark Matter in the universe remains unknown. Multiple astronomical measurements show that dark matter accounts for most of the matter of the universe. Imagine if the periodic table had a hole where Hydrogen should be, but astronomers could see giant clouds of an undiscovered element out in the Orion nebula. Then it would be an extraordinary time to be a chemist!
Similarly, imagine if every fermion had a boson partner and vice versa. In that case, nature would be a little more symmetric than what we have discovered so far. This prediction, called supersymmetry or SUSY, helps explain some of the holes in the standard model. One of the hypothetical new particles described by the SUSY theory could even be the missing dark matter particle. These "SUSY partners" must be heavy; otherwise, we would have discovered them already. Finding evidence of supersymmetry is one of the main goals of the big experiments at the Large Hadron Collider, such as the CMS experiment.
Einstein's theory of relativity tells us that matter and energy are related and can be exchanged. The LHC produces this kind of exchange when the energy of the high-speed proton collisions creates new particles. The most massive elementary particle we know about so far is the top quark, which has a mass similar to that of the element Rhenium, which has 75 protons and about a 100 neutrons in its nucleus. The LHC can, in principle, produce new particles more than ten times as massive as the top quark. Such particles would rarely appear in the data because in general, the larger the mass, the less likely particles would be produced.
Theories like SUSY can predict how new particles are produced in the LHC and measured in the CMS detector. Typically, these theories impose rules for producing new particles from ordinary matter, for example, that new particles are only produced in pairs. New particles can be unstable, decaying to lighter and lighter particles, and the standard model decay products are captured and measured in the CMS detector. However, eventually, many models of supersymmetry predict that the decay chain will end in a stable particle that would escape the detector unmeasured, resulting in a significant imbalance in where the particles are situated in the detector. This imbalance could be the signature for supersymmetry or dark matter particles produced at the LHC!
Figure 1: A graphical display of measurements made by the CMS detector. Looking in a two-dimensional projection along the cylinder of the detector, the trajectory of charged particles can be seen in the yellow lines emerging from the center. Streams of these particles are combined into jets grouped in the orange cones, and the size of their combined energy is shown as the sum of red and blue bars. The imbalance of their total momentum is seen in the purple arrow. Could this be a new particle exiting the detector without leaving a measurement, or is it just invisible standard model particles that were not detected, like neutrinos?
The CMS detector has amassed a large amount of data from collisions starting in 2016 and taking place through the end of 2018. This large data set allows CMS physicists to classify measurements according to interesting features in the collision. We focus on "jets," which are streams of particles that result when quarks or gluons are produced in the collision. Figure 1 shows an event with six jets. Summing their directions and momentum shows that they would be balanced by an undetected particle exiting the detector along the purple arrow labelled MHT. Now let us not get too excited yet, as neutrinos escaping the detector are also unmeasured and can sometimes give rise to the purple arrow. Neutrinos are known decay products of known elementary particles like the top quark, W boson and Z boson. If we consider a collection of data, we can do a statistical analysis to assign a probability of how much the data disagrees with these known processes. Figure 2 shows that very likely all the data are consistent with a combination of three known processes. In this way, we look for rare particles by very carefully measuring the standard model.
Figure 2: This histogram shows a chart of three known standard model processes and how many times they occur to give rise to a substantial momentum imbalance. The black points represent the number of measured collisions. The agreement between the measurement and the predictions for standard model processes is good.
Figure 3 is an example result for the production of gluinos, the SUSY partner of the gluon, from the proton proton collision. In this case, we presume the gluino decays into a pair of top quarks and the stable, lightest, SUSY particle (LSP), which escapes detection and causes momentum imbalance. This decay chain is simplified because the gluino decays only to a few particles and the LSP, but this encapsulates many common features of SUSY theories. Figure 3 shows the possible combinations of the gluino and LSP masses for this decay chain. The black curve separates mass combinations where this decay is highly unlikely from the combinations where this decay is still possible. Combinations with large gluino mass (towards the right) and large LSP mass (toward the top) are still possible..
Figure 3: This chart shows a region enclosed by the black line where the probability of new SUSY particles is low. The horizontal range gives the mass of gluinos, and the vertical range gives the mass range of the SUSY LSP. The range of colors shows the maximum allowed rate for the production of these particles based on our measurements.
However, the story does not end here! In planning for future theories and sharpening the focus of experimental results, Figure 3 is like a bookmark at the end of the chapter of the most current LHC data. More data will allow us to push our results to larger masses and rarer SUSY processes. The future of the LHC will offer a large increase in the number of proton collisions at a larger center-of-mass energy of 14 TeV, which will magnify the focus of missing momentum searches on new particles. The LHC will be able to collect the same amount of data shown here in just one year, and the CMS detector will be upgraded to sharpen measurements and narrow the focus on interesting collisions that could contain new particles. From the knowledge we have gained from the current data, we will plan and build new searches to discover new symmetries of the basic building blocks of matter.
Read more about these results in the CMS Physics Analysis Summaries:
- Log in to post comments