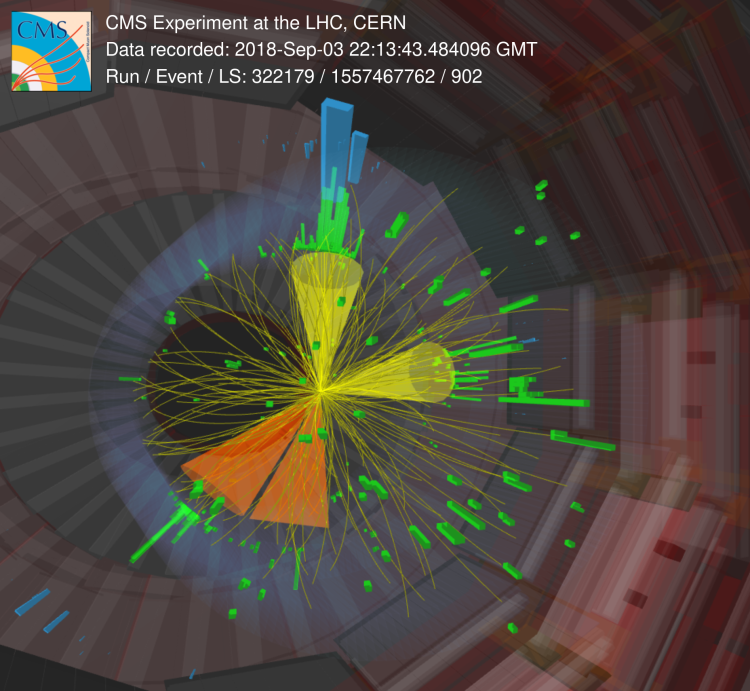
It is easy to recognize your friends in a street if they are standing or walking, but what if they are riding a supercar at a speed of 150 km per hour? In the Large Hadron Collider, if the energy of two partons from two colliding protons is about the mass of the Higgs boson, 125 GeV, a Higgs boson can be produced, almost at rest, so that its decay products are emitted back-to-back. This feature is imposed by a physics principle called conservation of momentum. Most of the Higgs boson candidates found in 2011 or 2012 and leading to its discovery were like this. If the partons carry much more energy, instead, the produced Higgs bosons can have a considerable momentum, and their decay products will be more collimated: higher momentum means higher collimation. Higgs bosons with a significant speed or, more precisely, with a high momentum in the plane perpendicular to the beams, the transverse momentum pT, are called Lorentz-boosted. Measuring their properties tests the existence of new phenomena in the scalar sector of the standard model (SM), the sector that deals with spin-0 particles, such as the Higgs boson itself. Measurements could highlight deviations in the production cross section of the Higgs boson, known with high accuracy in the SM. Furthermore, these measurements can shed light on the structure of the interactions of the Higgs boson with massive or strongly interacting particles and might uncover beyond-SM effects that are not revealed through inclusive measurements.
Boosted particles have been the subject of much interest among experimental particle physicists in the last few years. A pair of boosted particles, such as the W, Z or Higgs bosons, can arise from the decay of a new heavy resonance. A single boosted particle can also be produced together with a high-momentum jet. The decay products of the boosted particles are spatially close, forming a wide jet of particles. Dedicated techniques, based on the substructure of wide jets, are used to identify jets originating from the hadronic decay of Lorentz-boosted particles and distinguish them from the more usual “narrow” jets. Figure 1 displays a Lorentz-boosted Higgs boson decaying to a pair of close-by tau leptons (heavy siblings of electrons) from a collision event recorded in 2018.
Figure 1: A Lorentz-boosted Higgs boson decaying to a pair of close-by tau leptons, from a collision event recorded in 2018. The two hadronically decaying tau leptons associated with the decay of the Higgs are shown as two orange cones. The yellow cones represent jets that are produced in association with the Higgs boson. Try to rotate and zoom on this separate page.
CMS has just released the first measurement of the cross section of Lorentz-boosted Higgs bosons decaying to a pair of tau leptons, using all the proton-proton data collected during the LHC Run 2. To impose a boosted topology, the tau leptons are required to have an angular separation smaller than 0.8, corresponding to Higgs bosons with transverse momenta larger than 250 GeV. A dedicated algorithm to select a pair of close-by tau leptons is necessary to maintain a high signal selection efficiency. Given their high masses, tau leptons can decay to lighter leptons (electrons and muons) or to hadrons, such as pions. Four final states, including hadronic and leptonic tau decays, are considered: μτh , eτh , eμ, and τhτh, where τh denotes the products of hadronic tau decays. A multiclass neural network is used to distinguish the signal from backgrounds, such as boosted Z bosons decaying to pairs of tau leptons or misidentified multijet events. The events are categorized according to their similarity to the signal or the backgrounds, and according to the transverse momentum of the Higgs candidate, pTH.
After analyzing the data, the product of the Higgs boson production cross section and the branching fraction to a pair of tau leptons is measured to be 1.64-0.54+0.68 times the SM expectation, consistent with the SM prediction within one standard deviation.
The Higgs boson differential cross section as a function of pTH has also been measured and compared, in each transverse momentum bin, with the corresponding SM prediction. Significant deviations can be a smoking gun for physics beyond the SM. Figure 2 compares the measured pTH distribution with the SM calculations: no deviations are seen, within the measurement uncertainties. These results show that the supercar-riding Higgs bosons behave as expected in the SM. The current uncertainties still leave room for possible beyond the SM effects, to be further scrutinized by analyzing more data.
Figure 2: Observed and computed Higgs boson differential cross sections versus transverse momentum. The Higgs signal is split into two classes, the first corresponding to gluon-gluon fusion (gg→H) investigated with two event generators (POWHEG and NNLOPS), and the second including vector boson fusion (VBF), associated Higgs boson production (VH), and Higgs boson production associated with a pair of top-antitop quarks (ttH).
Read more about these results:
-
CMS Physics Analysis Summary (HIG-21-017): "Measurement of the highly Lorentz-boosted Higgs boson cross section in the decay mode of a pair of leptons in proton-proton collisions at 13 TeV"
-
Display of collision events: CERN CDS
-
@CMSExperiment on social media: LinkedIn - facebook - twitter - instagram
- Do you like these briefings and want to get an email notification when there is a new one? Subscribe here