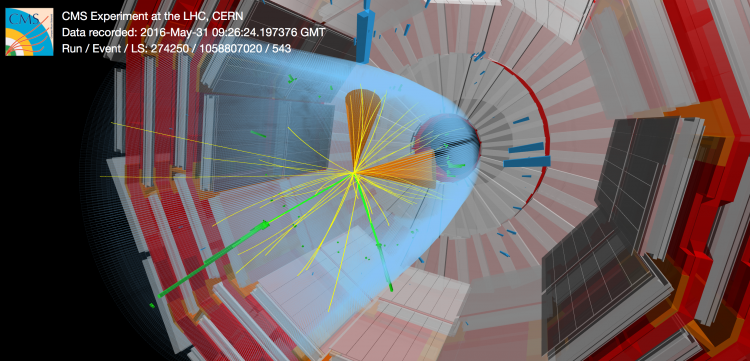
The CMS collaboration has carried out for the first time a direct search for a Higgs boson decaying into charm quarks, a vital step in understanding the Higgs particle and the Standard Model.
The discovery of a Higgs boson by both the ATLAS and CMS experiments in 2012 confirmed the electroweak symmetry breaking mechanism, the process through which elementary particles gain mass via interaction with the Brout-Englert-Higgs field. The mass of the Higgs boson is measured to be mH = 125.26±0.21 GeV, and its decays in γγ, ZZ, WW, bb, and ττ modes have been observed. All measured properties are within the uncertainties consistent with the predictions of the Standard Model.
Notwithstanding the progress since discovery seven years ago, there remains much to be learned about the properties of the Higgs particle. One of the highest priorities of the LHC physics program is the measurement of the couplings of the Higgs boson to other Standard Model particles. Physicists are still puzzled why the Standard Model has three generations of matter particles (also called fermions). Up to now, the coupling of the Higgs boson to quarks has directly been studied in connection to fermions from the third generation. In 2018, both the ATLAS and CMS Collaborations reported observations of the Higgs boson produced in association with a top-quark pair. This result represented the first direct observation of the Higgs boson coupling to quarks. With the previous discovery of the Higgs boson decays to tau leptons and subsequently to bottom quarks, all the Higgs couplings to third-generation fermions have been measured and found statistically consistent with the predictions of the Standard Model. However, it is not possible to completely characterize the Higgs boson and its role in the Standard Model without assessing all its possible coupling to the known particles, including all other quarks.
It is of utmost importance to investigate whether the spontaneous symmetry breaking mechanism is the unique source of mass generation in the Standard Model. The next goal in the characterization of the Higgs boson properties is the measurement of the coupling of the Higgs boson to second-generation quarks. The coupling can be significantly modified by new physics phenomena, beyond the Standard Model.
In the Standard Model, the coupling of the Higgs boson to any particle is proportional to the mass of the particle itself. With a mass of approximately 1.3 GeV, the charm quark is roughly 130 times lighter than the top quark and about three times less massive than the bottom quark. Given the moderate mass of the charm quark, the expected fraction of Higgs boson decays into charm quarks is predicted to be quite modest when compared to bottom quarks. Moreover, the experimental difficulties in identifying charm quarks at the LHC make this search a real challenge.
CMS physicists decided to use an analysis strategy that targets events in which the Higgs boson is produced in association with a W or a Z boson, as depicted in Figure 1. The most important background process at the LHC with a similar signature is the production of a Z boson in association with two additional charm quarks.
Figure 1: Feynman diagram of the signal process targeted in the analysis. Two interacting quarks contained in the colliding protons give rise to a virtual Z or W boson, that subsequently emits a Higgs boson. In turn, the Higgs boson should very rarely decay into a pair of charm quarks.
The approach exploits two different regimes of the Higgs boson transverse momentum. Depending on the transverse momentum of the Higgs boson, its decay products can be more or less collimated. Moreover, each quark will give rise to a cascade of particles called a jet, that provides information about the initial direction and momentum of the quark that originated it.
To search for the direct decay of the Higgs boson decaying to charm quarks, CMS physicists look for two different signatures: two separate charm quark jets, or for a unique "fat” jet of particles. Such a fat jet has a larger size and can contain both the charm and anti-charm quark from the Higgs boson. This distinction helps to identify all the possible scenarios accurately and maximizes the analysis sensitivity — figures 2 and 3 show how these two different topologies appear in the CMS detector.
Figure 2: Representation of a collision recorded by the CMS detector that features a Z boson decays to an e+e- pair and two charm jets that could be from the Higgs boson. The red and blue towers represent the energy deposits in the CMS electromagnetic and hadronic calorimeter, respectively, while the green lines represent the tracks of the charged particles as reconstructed by the CMS tracker. The yellow cones identify the jets assigned to charm quarks. The purple arrow indicates the missing momentum in the event carried out by undetectable particles such as neutrinos.
Figure 3: Representation of a collision recorded by the CMS detector that features a Z boson decays into neutrinos and a “fat” jet consistent with two charm jets, that could be from the Higgs boson. The red and blue towers represent the energy deposits in the CMS electromagnetic and hadronic calorimeter, respectively, while the green lines represent the tracks of the charged particles as reconstructed by the CMS tracker. The yellow cones identify the jets created by charm quarks. The purple arrow indicates the missing momentum in the event carried out by undetectable particles such as neutrinos.
Eventually, to recognize the selected jets as produced by charm quarks and discard those originated from other flavor quarks, dedicated algorithms based on advanced machine learning techniques have been deployed. In the case of jets from one charm quark, a Deep Neural Network is trained to identify jets of particles that originated from charm quarks. The neural network exploits information like the direction and energy of the jet, the information relative to the charged particle tracks inside the jet and the presence of vertices found displaced with respect to the origin of the jet of particles. Such displaced vertices are a sign of the possible presence of particles that contain charm quarks and that travel for a few tenths of a millimeter from the proton-proton collision point. The output of the charm quark identification algorithm is combined with other observables in another neural network to perform the final isolation of the signal from the background. In the “fat jet” topology, instead, a novel method based on a complex Deep Neural Network architecture is trained to directly distinguish Higgs bosons decaying into a couple of charm quarks from the main background processes. The double-charm neural network uses similar information but also uses the internal structure of the “fat” jet and global information on the collision, including on other particles that are present. The output of the final neural network is used for the final signal extraction.
The final results set limits on the coupling between the Higgs boson and charm quark, improving the previous analysis sensitivity to the charm decay of Higgs bosons by more than a factor four. We expect significant further improvements in the sensitivity to the decay of the Higgs boson to charm quarks after fully exploiting the available LHC Run-2 data. With the ongoing and foreseen detector and accelerator upgrades in LHC Run-3 and, after that, High Luminosity LHC.
Read more about these results in the CMS Physics Analysis Summaries:
- Log in to post comments