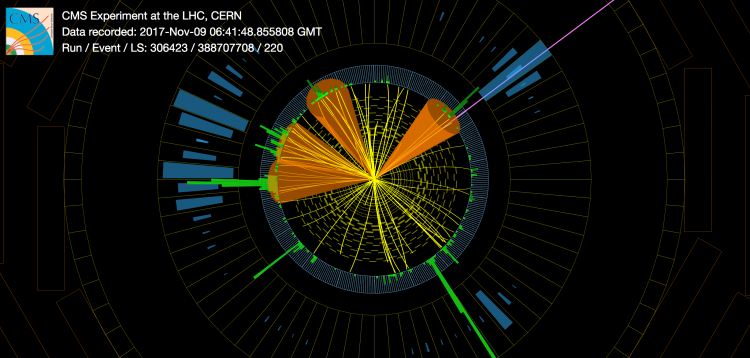
New physics may show up at the LHC in the form of yet unobserved particles which travel inside the detector before decaying, far from the point in space where they were produced. If these particles decay to photons, at CMS we can use the short but measurable time these photons move through the detector to identify them. These techniques are now used by the CMS collaboration for a search for delayed photons.
The enigmatic Higgs boson, a particle predicted by the Standard Model, was discovered at the LHC in 2012, and its properties have been shown by the CMS experiment to match a wide range of Standard Model predictions with increasing precision ever since. However, conceptual shortcomings that cannot be explained within the Standard Model itself fully support the expectation that physicists should find evidence for physics beyond the Standard Model. These searches for physics beyond the Standard Model become more sensitive as experiments continue to collect an ever-increasing data set at the LHC. Searches for new particles which travel inside the CMS detector and then decay, far from the point where the LHC protons beams collide, are one of the most promising paths to an early discovery. These new particles, called long-lived particles, usually decay to Standard Model objects such as leptons, photons and collimated jets of hadrons, and are predicted in scenarios where the couplings between the new and known Standard Model particles are very small as well in theories which describe the existence of dark matter in the universe. Long-lived particles are particularly well motivated by the fact that the LHC experiments have so far not observed new particles that decay close to the point in space where they are produced.
The ability to measure the time required for long-lived particles to travel inside CMS before decaying (called proper lifetime), has revolutionized our search strategies and experimental techniques. The search described here exploits a novel technique to detect, in CMS, photons from the decay of long-lived particles produced by 13 TeV proton-proton collisions during LHC Run2. Here we analyze the data sets collected in 2016 and 2017 to search for a neutralino (): a neutral, massive long-lived particle, predicted by many theoretical models which are based upon a new kind of symmetry in nature called supersymmetry. The neutralino travels a distance in the CMS detector from a few millimeters up to several meters before it decays to a photon and a stable massless gravitino (another particle predicted by supersymmetric models). The photon’s energy and angle are measured precisely in the CMS detector, while the gravitino, that interacts only weakly with normal matter, escapes undetected, leaving behind the characteristic signature of an apparent energy imbalance transverse to the beamline.
Figure 1: A sketch of a photon from a Standard Model process is shown on the left. It is compared with a similar sketch of a delayed photon from the decay of a long-lived neutralino on the right. The latter reaches the detector delayed compared to the left one. This delay can be measured with the electromagnetic calorimeter (ECAL).
As sketched in Figure 1, photons produced in the decay of a long-lived neutralino reach the detector at a later time than a prompt photon directly produced at the interaction point by conventional Standard Model processes.A signal from delayed photons is identified here exploiting one of the main features of the CMS apparatus: its lead tungstate crystal electromagnetic calorimeter (ECAL): a detector devoted to the identification and precise measurement of neutral particles such as photons. The performance of the ECAL in measuring the energy of photons with an uncertainty (resolution) of order 1% has been one of the key ingredients in the observation of the Higgs boson by CMS. But this is not the end of the story. The ECAL crystals are also very fast detectors, which means that in addition to the energy deposited by a photon hitting the detector, they also provide a measurement of the time when this energy arrived with respect to the time of the proton-proton collision, which is known to a great accuracy. The capability of the ECAL to measure the photon arrival time with a resolution better than 100 ps allows us to identify late-arriving photons, which originate at a point which is separated from the ``interaction vertex” where the two protons in the LHC collide.
Separating out a sample of events with this unique signature from the Standard Model backgrounds requires specialized filtering algorithms, which include dedicated photon reconstruction and identification criteria. To identify the signal (represented by events with a neutralino decay), we select events with at least one photon that enters the ECAL at an angle that is not orthogonal to the surface of ECAL, consistent with a delayed signature, as shown in Figure 1. CMS physicists exploit the striking simultaneous combination of this feature and the information of their time of arrival at the ECAL to identify the potential signal candidates. Signal events are also expected to have significant amounts of missing energy in the plane orthogonal to the direction of the colliding beams, because of the gravitino, the lightest supersymmetric particle, which could have escaped the detector volume undetected after being produced in association with the photon in the decay of the neutralino.
After selecting events with delayed photons and, at the same time, a large amount of missing energy in the event together with many jets, the data does not significantly deviate from the background expectation. The search result is thus interpreted in terms of upper limits on the neutralino production cross section (a measure of the probability of producing them in a collision) for a specific theoretical model prediction assuming supersymmetry. Figure 2 represents an example of a candidate event selected from the data collected in 2017.
Figure 2: A display of a collision recorded by the CMS experiment in 2017 which shows a signature compatible with that expected for a signal event in this analysis. The purple line represents the direction of the missing energy and the high green tower on the bottom-right of the view is the photon in the event. The photon arrived at the ECAL 1.91 nanoseconds after the proton-proton collision in the LHC occurred.
Figure 3 shows how sensitive the CMS experiment is to delayed photons in terms of the mass of the neutralino and proper lifetime. The values of the masses and lifetimes to the left of the black curve in the figure are those excluded by this search, leaving room for them to hide only at higher masses and/or longer lifetimes. This is the first result on this kind of signature at LHC Run 2, and it extends significantly the knowledge we had on long-lived neutralinos from previous searches. Clearly the better the time resolution of our detector, the shorter the lifetime of the long-lived particle that we can probe. These promising results represent the starting point and lay the foundation for searches that will exploit the future upgrades to the CMS detector. These upgrades include a new thin layer of fast and bright scintillating crystals that emit light when particles pass through them and are situated just in front of the present ECAL. The upgrade will provide improved time measurements for photons which are an order of magnitude more precise than in the present detector, and which will be one of the key ingredients of many other long-lived particle searches as well as other analyses performed by CMS in the future.
Figure 3: The results of the delayed photon search. The red dashed region, and the black and red lines draw the "limit" of the knowledge we obtained about long-lived neutralino with this search. The values of neutralinos` masses (on the x axis) and lifetimes (on the y axis) on the left of these curves can be considered excluded by this search. The lifetime is indicated in this figure as "ctau", the time measured by a clock traveling with the particle, thus independent of its actual speed in the detector, according to the theory of relativity. As visible, this search significantly extends previous results, which are here represented by the azure and the green borders.
Read more about these results in the CMS Physics Analysis Summaries:
- Log in to post comments