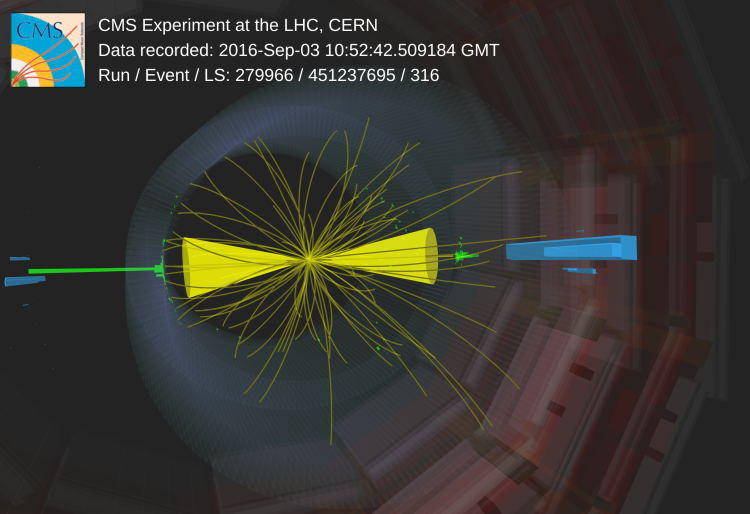
Among all the elementary particles, quarks and gluons (partons) are the only ones that cannot be observed in isolation. In high-energy collisions, such as those occurring at the Large Hadron Collider (LHC), partons undergo a few stages between their production and reaching our detectors. Governed by quantum chromodynamics (QCD), they initiate a parton shower process, which generates a cascade of radiation, so that a lot more quarks and gluons are produced by the end of this stage. Eventually, this splitting ceases and the partons combine to form composite particles called hadrons. Protons and neutrons are familiar examples of hadrons. This stage, where color is confined, is a unique feature of the strong force: partons cannot be observed individually. The process of confinement is difficult to describe by theory models and challenging to study experimentally. Jets measured in collider experiments provide key information to probe the time evolution from partons to hadrons. Theorists suggested that the energy and angular correlations of particles inside a jet may provide valuable insights into the journey that turns partons into hadrons.
Such correlations can be studied through a set of observables called energy correlators. E2C is the simplest energy correlator: the angular distances between any two particles inside a jet, xL, are plotted in a distribution, with weights proportional to their energy fractions within the jet. The E3C observable is similar to E2C, with the angular distance being calculated among three particles instead of two, and the longest being taken. These observables are very effective in separating the different stages of the parton to hadron evolution within the distribution. The small and large xL regions reflect the interactions between hadrons and between partons, respectively, and are expected to have different behaviors.
The CMS collaboration recently reported a measurement of the E2C and E3C observables. Figure 1 shows the measured E2C distribution. Two distinctive behaviors are observed that could be associated with two stages of jet formation. The parton shower splitting follows perturbative QCD and is rather wide-angled (which means that there is a large energy exchange), producing partons separated by large xL values. The particles stay apart after hadronization and contribute to the large-xL region. According to parton level theoretical calculations, the corresponding E2C distribution should have a falling behavior, as we see on the right-most part of the figure. Therefore, that region can be assumed to be dominated by parton interactions. The additional soft hadrons produced in the hadronization stage have lower energy exchanges and are separated by smaller angles. According to theory, the hadron-dominated part of the E2C distribution should have a rising behavior, as we see in the small-xL region of the figure. The two patterns are separated by a transition, corresponding to the parton-to-hadron confinement transition.
Figure 1: The measured E2C distribution, in several jet transverse momentum ranges.
How does the confinement behavior change with the jet transverse momentum? The answer can also be seen in Fig. 1, which shows, through the animation, how the measurement changes when we vary the jet momentum range. As the momentum increases, the boundary between the two regions moves to lower xL values. This can be explained qualitatively: a parton produced with a higher momentum is more likely to undergo additional splittings before the process stops. More splittings means a wider parton-shower region, so that the boundary gets pushed to the lower xL region.
This analysis also provides a measurement of the strong coupling constant (αS), a fundamental parameter of the standard model that determines the strength of the strong interaction processes and affects the cross sections of almost all the physics processes at the LHC. Previously, the inner structure of jets was rarely used to extract αS. The new CMS measurement derives it from the distribution of the E3C/E2C ratio as a function of xL, and its variation with the jet energy, shown in Fig. 2 for several jet momentum ranges. The variation of the slope of the ratio in the perturbative region indicates that S decreases with the energy scale, reflecting the well known asymptotic freedom of QCD: quarks behave as free particles when the energy is high enough.
Figure 2: The measured E3C/E2C ratio in several jet transverse momentum regions.
The E3C/E2C ratio measurement, together with the most precise theory predictions, allows one to extract αS. It is determined to be 0.1229 with a precision of 4% at the energy scale of the Z boson mass, consistent with the world average value and becoming the most precise αS measurement using jet substructure.
The three PhD students who played a key role in the measurement, Chenfeng Lu, Yulei Ye, and Zhen Lin, expressed their excitement about the implications of this work: “Studies of jet substructure have a great potential to improve our understanding of fundamental features of QCD”.
Read more about these results:
-
CMS Physics Analysis Summary (SMP-22-015): "Measurement of energy correlators inside jets and determination of the strong coupling constant"
-
@CMSExperiment on social media: LinkedIn - facebook - twitter - instagram
- Do you like these briefings and want to get an email notification when there is a new one? Subscribe here