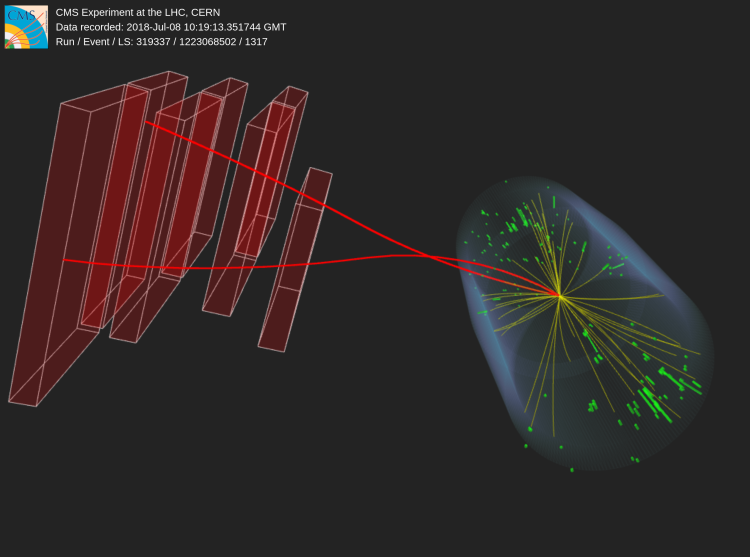
Experimental particle physics is like a game of hide and seek. Physicists endeavor to seek what is hidden by exploring all the possibilities. The hidden truths, which interpret the rules of nature, lie within elementary particles and how they interact with each other. To reveal the truths, physicists build colliders to smash particles at high energy, creating possibilities for new particles to emerge. Usually, those new particles are good at hiding. They are invisible to us as they may have a very weak interaction with the known particles, leaving no trails in the detector. They may also be unstable and decay into other products before the detector can even see them.
Over decades, physicists have found that an effective way to detect hiding particles is to search for their visible “legs”, outside their hiding place. These “legs” are known particles produced in the decay of the hiding particle. Many important discoveries have been made in the past using this method. For example, by scanning the production of two known particles, we have discovered the J/Ψ and Y mesons, and the Z and Higgs bosons.
The CMS experiment is particularly good in measuring pairs of muons, which we can use as visible legs to search for new particles. The benefit of this approach is that muon pairs (dimuons) lead to well-defined signatures of the production of new particles, seen as high-resolution peaks when reconstructing the mass of the muon pair. Until recently, the CMS collaboration had only searched for dimuon resonances of masses above the Y states, at around 10 GeV. We have now searched for muon pairs from decays of new particles lighter than 10 GeV. Our good companions, the theorists, have predicted the possible existence of new particles that could produce such signatures. Since their production is a very rare process, these light particles could have evaded searches in previous experiments; at the LHC, thanks to the very large collision energies, they could become visible. One of the most exciting candidates is the dark photon, a new gauge field predicted by the dark matter theory that interacts with standard model (SM) particles similarly to the well-known photon. Another candidate is a pseudo-scalar particle predicted in a theory that extends the SM, called “two-Higgs-doublet model with an extra complex scalar singlet”. Both these candidates are potentially produced in the proton-proton collisions, subsequently decaying to two muons.
Figure 1: Low-mass dimuon detected by CMS. The two red lines represent the two muons. Other lines represent charged-particle tracks originating from the same collision. The interactive event display is available also on this separate page to zoom and rotate.
A major challenge in searching for such low-mass new particles is the low momentum of their decay products. Low momentum muons are profusely produced, leading to event rates that are too high to be handled by the standard CMS trigger and data acquisition systems. To overcome this limitation, we have developed an innovative approach, known as the “data scouting” trigger system, which directly reconstructs the event data in the trigger system. By only storing the essential information of each event, this approach reduces the amount of data to be stored, thereby significantly increasing the rate of events that can be processed. The data scouting technique was used in Run-2, leading to a data sample corresponding to an integrated luminosity of almost 100 fb-1. The technique used to search for the signal peak is relatively simple. If the new particle is produced and immediately decays into two (oppositely charged) muons, then it should be visible as a sharp resonance peak in the dimuon mass distribution, on the top of a smooth continuum produced by other (already known) processes. A dimuon event reconstructed in the CMS detector is shown in Fig. 1.
Figure 2: Background-only and signal-plus-background fits of the mass distribution of dimuons with transverse momentum exceeding 35 GeV, in a window around 2.4 GeV, using the 2018 data. The lower panel shows the pull distribution: the difference between the data and the background function, divided by the statistical uncertainty of the data.
We scan the dimuon mass distribution and, for each mass value, we evaluate if the data calls for the presence of a “bump”. The search was performed using the 2017 and 2018 scouting data, down to lower masses than ever before. Apart from a hint that something might be starting to show up at around 2.4 GeV, when we select dimuons of transverse momentum exceeding 35 GeV, as shown in Fig. 2, we see that the dimuon mass distribution can be described by a smooth continuum. The absence of clear signals of new particles can be used to set limits on how likely it is for a particle to exist, at that mass, as shown in Fig. 3. These limits are used to constrain the hypothetical theory models and also to adapt future data analyses aimed at finding new particles.
Figure 3: Observed (solid line) and expected (dashed line) model-independent upper limits on the signal cross section times the branching fraction to a pair of muons, as a function of the dimuon invariant mass: higher product values are excluded. The green (yellow) band is the 68% (95%) confidence level interval around the median expected limit.
The data scouting method is clearly a very successful tool in searches for low-mass particles, increasing the search sensitivity while limiting the data size. CMS is using an improved version of the data scouting technique in the LHC Run 3, which started in 2022. With more efficient triggers and larger data samples collected, we will have broader and more sensitive searches of dimuon resonances potentially generated from the dark photon or other new particles. If there are resonances at low dimuon masses, they will become more significant and we might be able to observe them!
Read more about these results:
-
CMS Physics Analysis Summary "Search for prompt production of a GeV scale resonance decaying to a pair of muons in proton-proton collisions at 13 TeV"
-
@CMSExperiment on social media: facebook - twitter - instagram
- Do you like these briefings and want to get an email notification when there is a new one? Subscribe here