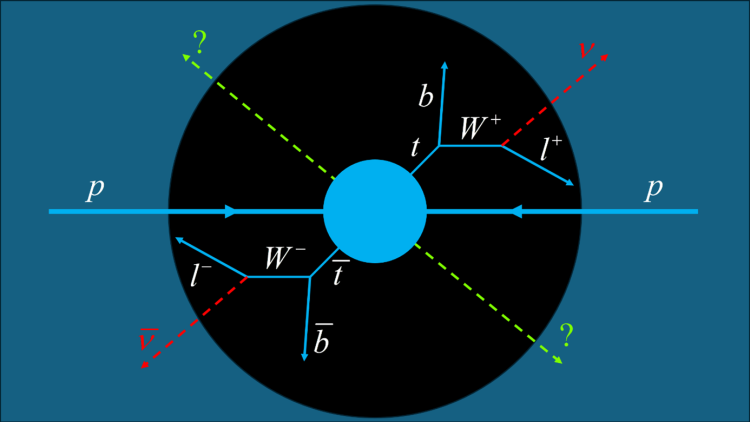
Top quarks are the heaviest known elementary particles, as heavy as a tungsten atom. In high energy proton proton collisions at the LHC about 10 pairs of a top quark and its antiparticle are produced every second. But they are not here to stay: top quarks decay almost immediately, after traveling on average only about a tenth of the radius of a proton. In about one third of cases the top quark decays into a bottom quark, a charged lepton (electron, muon, or tau) and a neutrino. Since these neutrinos leave the detector undetected, a fair fraction of the energy of the top quark is invisible – as invisible as new particles might be which could be created in the LHC collisions. Actually, in many extensions of our current standard model of particle physics, these new particles are produced together with charged leptons and bottom quarks, creating a very similar signature to that of top quark pairs in the detector. It is therefore very important to measure the amount of energy leaving the detector unnoticed in top quark pair events.
How can one measure the energy of undetected particles? We know that the total momentum transverse to the direction of the colliding protons is zero before the collision and therefore must be zero also in the final state. What is missing is called “missing transverse momentum”, or “missing pT” for short, and ideally represents the combined transverse momentum of the two “prompt” neutrinos from the decays of the top and the anti-top quarks. In this way we can measure the invisible particles from the momentum imbalance of the visible ones. Unfortunately, it is not that simple. Any mismeasurement of any visible particle in the detector can add to the missing pT. And the decays of the bottom quarks may contain further neutrinos. In his PhD thesis, Danilo Meuser of RWTH Aachen University has developed tools to tackle these problems: a deep neural network to correct for detector mismeasurements, and a procedure to determine from the measured missing pT the combined transverse momentum of the prompt neutrinos, pTνν. This allowed Danilo to measure, for the first time, the cross section to produce a top quark pair as a function of the combined transverse momentum of the two prompt neutrinos from the top decays. The analysis effort was supported by postdoc Marius Teroerde and towards publication by postdoc Sandra Consuegra Rodriguez.
Figure 1: The measured signal cross section (black markers) as a function of pTνν. The theoretical predictions from POWHEG+PYTHIA (dark red), POWHEG+HERWIG (orange), MC@NLO+PYTHIA (purple), and the fixed-order NLO (light blue) and NNLO (brown) calculations are compared to the measurement. The total (statistical) uncertainty on the measurement is shown as an orange (dark gray) band. The bottom panel shows the ratio of each theoretical prediction to the measurement.
This brand-new CMS result was presented for the first time at ICHEP 2024 . Figure 1 shows the cross section as a function of pTνν. The measurement (black markers) reaches out to momenta of several hundred GeV. It is compared to different state-of-the-art Monte-Carlo simulations and to theory calculations at the highest currently achievable accuracy of next-to-next-to-leading order (“NNLO”) as well as one order less (“NLO”) for top quark pair production. The measurement shows that, within uncertainties, the combined neutrino momentum is correctly described by these predictions. The cross section was also measured as a function of the angle between the direction of the combined neutrino momentum and the nearest charged lepton as well as two-dimensional as a function of both variables, with similar conclusions. This result is an important test of the standard model in a very interesting, but so far unexplored region. We are now better prepared to look for deviations from the standard model due to new physics. As LHC data at higher center-of-mass energy arrive, we expect more events at large pTνν values. They will either prove the standard model in the extreme corners of the phase space, or give hints for new particles that could help resolve some of our unanswered questions about Nature.
Edited by: Muhammad Ansar Iqbal and Abideh Jafari
Read more about these results:
-
CMS Physics Analysis Summary (TOP-24-001) " Measurement of the dineutrino system kinematics in dileptonic top quark pair events in pp collisions at sqrt(s) = 13 TeV"
-
Display of collision events: CERN CDS
-
@CMSExperiment on social media: Bluesky - Facebook - Instagram - LinkedIn - TikTok - Twitter/X - YouTube