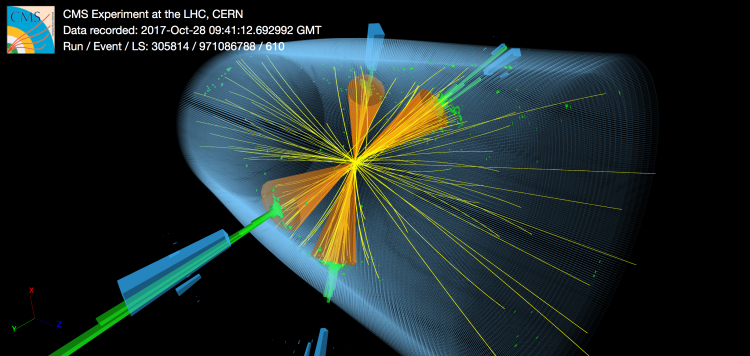
At the EPS-HEP conference this week the CMS collaboration presents new results of a powerful search that is motivated by a variety of new physics models that predict a signature of a bump in the production of the most frequently produced particles at the LHC, quarks and gluons. All the available collision data collected in run 2 of the Large Hadron Collider are used. The much larger dataset together with a new analysis methodology significantly extends the discovery potential of previous searches.
The field of Particle Physics is currently facing an extremely puzzling success: there is a theory, the Standard Model, that describes particles and their interactions. The Standard Model has been very successful in predicting most observed and measured physical phenomena at particle physics experiments in the past fifty years, including those related with the Higgs boson discovery in 2012 at the Large Hadron Collider (LHC). Despite these achievements, the Standard Model is not able to provide answers to fundamental questions like: What is the nature of Dark Matter and Dark Energy composing 95% of the universe? What is the source of the dramatic matter-antimatter asymmetry in the universe? Do all forces become one at a higher scale, and how is gravity incorporated? Are there new, additional, symmetries in nature? Due to these unanswered problems, the Standard Model is widely viewed as only an incomplete “effective” theory, which must be extended to achieve a description of particles and their interactions at all energies. One of the most important jobs of the physicists at the LHC is the exploration and exploitation, experimental and theoretical, that can lead to even partial answers to these questions.
The LHC is well positioned to address these fundamental questions: it provides proton-proton collisions at 13 TeV, the highest energy to date, enabling experiments to test and probe the Standard Model at unprecedented energies and with high precision. LHC experiments have an enormous potential for discovering physics beyond the Standard Model, with an ability to test a variety of plausible extensions to it, like extra dimensions, new gauge interactions including those between Dark Matter and Standard Model particles, new fermion families, new massive neutrinos and many more.
Many of the extensions of the Standard Model predict resonant production of new particles that would then decay to a pair of quarks or gluons. Quarks and gluons cannot exist by themselves and manifest themselves in the CMS detector as collimated sprays of particles along the initial parton direction, that are called hadronic jets. In this case, the physics signature is a collision yielding two jets (also called dijet) in the final state. New physics in this final state would manifest itself with a rather simple experimental signature: as a localized (narrow) or spread out (broad) enhancement of the SM prediction of the invariant mass distribution of the dijet system, as shown in Figure 1. A dijet resonance search is a very generic and model-independent method of targeting a variety of many new physics models.
Figure 1: Dijet mass distribution for the experimental data (black dots and red line) with some hypothetical new physics signals (coloured dashed lines) displayed.
What is critical in a dijet resonance search is to be able to predict the Standard Model background in the most accurate and unbiased way possible. The better the Standard Model background is predicted, meaning having smaller “systematic” uncertainties, the easier it is to discover even tiny signs of new physics. In this analysis, CMS physicists developed a new more robust method to predict the SM background. The strategy involved using experimental data where new physics is already known not to be present to best estimate the Standard Model background. This new method gives smaller uncertainties on the background estimate compared to the strategies used up to now for this kind of analysis.
This results in a significant enhancement of the discovery potential, especially for broader resonance searches which are of greater interest when hunting for a new force mediator connecting Dark Matter and Standard Model particles. In the absence of any excess of events above the Standard Model expectation, CMS physicists set upper limits on the production probabilities, called production cross sections, of these new resonances. These cross section limits can be converted to lower limits on the mass of new possible particles, assuming specific new physics models. The fact that the data is consistent with the Standard Model predictions means that if these new resonances exist, they are produced less frequently than what we can currently detect, or the particles have a higher mass than the LHC has the sensitivity to see with this dataset. This is depicted in Figure 2, where given specific new physics models, all resonance masses below the crossing of the theoretical cross sections (lines) with the measured cross section (coloured points) are excluded.
Figure 2: The observed (coloured points) upper cross section limits compared to predicted cross sections for a variety of new physics models like string resonances, excited quarks, axigluons, colorons, scalar diquarks, color-octet scalars, new gauge bosons W’ and Z’ with SM-like couplings, dark matter mediators.
Since LHC is the highest energy collider currently in operation, it is crucial to pay special attention to the highest dijet mass events where first hints of new physics at higher energies could start to appear. Figure 3 shows a collision recorded by the CMS experiment, with all the characteristics concerning jet masses, momenta, and angles, of one of our two highest dijet mass events. This collision contains four small cone-size hadronic jets in the final state, which in turn can be paired in one wider jet. This specific collision, with an invariant mass of the two wide-jets of 8 TeV, is quite unusual because the mass of each of the two wide jets has the same value of 1.8 TeV allowing for the possibility that it originates from a particle that in turn is decaying to a pair of dijet resonances.
Figure 3: Three-dimensional display of the event with the second highest dijet invariant mass at 8 TeV. The display shows the energy deposited in the electromagnetic (green) and hadronic (blue) calorimeters and the reconstructed tracks of charged particles (yellow).
This unusual high dijet mass event could likely be a collision created by the Standard Model background or possibly the first hint of new physics, but with only one event in hand, it is not possible to say which. The foreseen and planned new data-taking period in the near (Run 3) and longer-term future (High Luminosity-LHC), will increase the amount of data the CMS experiment will collect and analyze by factors of ten. This will allow CMS physicists to continue their hunt for such new particles. Along with planned improvements of our detectors and advancements in our analysis methodologies, future data will give us a chance to answer as to whether this exciting event is new physics or not, and hence make the near and long term future highly exciting!
Read more about these results in the CMS Physics Analysis Summaries:
- CMS-PAS-EXO-19-012: A search for dijet resonances in proton-proton collisions at sqrt(s)=13 TeV with a new background prediction method
- All CMS preliminary results
- All CMS results
- Log in to post comments