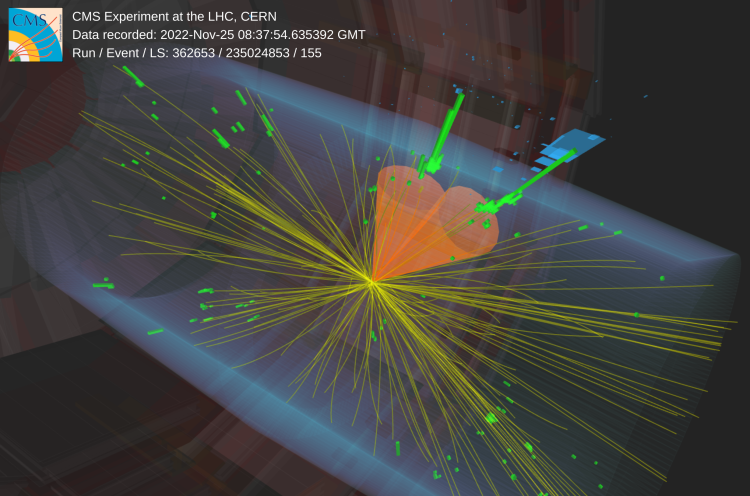
The discovery of the Higgs boson represents a significant breakthrough in understanding the universe’s fundamental structure and dynamics. Despite this achievement, numerous profound mysteries persist, namely what is the nature of dark matter, why the universe mostly consists of matter and hardly any antimatter, and why the measured Higgs boson mass is a finite number although calculations based on the standard model of particle physics (SM) lead to extremely large values. To address these fundamentally important questions, physicists at the LHC have conducted extensive searches for the signs of new theories beyond the standard model of particle physics. Traditionally, these searches have been mainly for new particles that decay immediately after their production. However, there has been a paradigm shift in recent years that focuses more on long-lived particles (LLPs) that travel measurable distances before decaying.
LLP signatures naturally appear in many well-motivated theoretical scenarios that can be the key to resolving the critical puzzles in physics. An important example is the case where there is a “hidden” or “dark” sector that exists, as also presented here, in parallel to the known particles of the SM. The dark sector can be a place where dark matter resides, and can also help explain the finite Higgs mass. It comprises particles that do not respond to SM forces but have their own unique interactions. In fact, the dark sector interacts only weakly with the known particles through possible “portals”. As pointed out by the Nobel laureate Frank Wilczek, the Higgs boson is a perfect candidate to act as a window to this dark sector that can elude detection otherwise. In this case, the Higgs boson can decay to the new particles existing in the dark sector, and these new particles can decay back to the known particles through the Higgs portal. Since the Higgs portal interactions are usually very weak, these decays can be “slow”, allowing the new particles in the dark sector to travel a significant distance before decaying into known particles, thereby manifesting as LLP signatures.
Figure 1: Left: The Higgs boson acts as a portal between the standard model sector and hidden/dark sectors, which can contain dark matter particles. Right: The diagram showing the process where a Higgs boson is produced at the LHC and decays to long-lived particles in the hidden sector, each of which further decays back to standard-model particles.
Detecting such a signature in the LHC experiments poses immense challenges. Due to the Higgs portal interaction, the new LLPs in the dark sector prefer to decay to bottom quarks that are relatively heavy. The bottom quarks then hadronize into showers of particles – called jets – originating from the point that is displaced from the point of collision. The bottom jets produced through standard model processes are already complicated objects, the displaced bottom jets from LLP decays are even more difficult to reconstruct and identify. Moreover, the collisions at the LHC produce a vast number of jets through strong interactions, creating an overwhelmingly large background source that makes it difficult to identify the rare decays of the Higgs boson to new LLPs from tens of billions of background events.
These challenges call for breakthroughs in our experimental techniques. Recently, CMS reported the result of a new search for LLPs decaying to displaced jets using the data collected in 2022, the first year of the LHC Run 3. A set of novel techniques have been developed and employed in this search. “We really pushed the boundaries of what we can do at CMS to probe challenging signatures of long lived particles.” Says Jingyu Luo, a postdoc at Brown University who leads this new search and the development of the new techniques. First of all, in this new data taking period of the LHC, new displaced-jets triggers have been deployed targeting the low-mass LLPs like those arising from the decays of the Higgs boson. Triggers are algorithms that operate in real time for data acquisition, selecting roughly 1000 events per second from tens of millions of events to save for future analyses. The events that don’t pass the trigger selections are lost forever, so it’s extremely important to have trigger algorithms that are efficient for the targeted signatures. A factor of 4 to 17 improvement in trigger efficiencies has been achieved with these new Run-3 triggers, allowing more signal events to be collected even with a much smaller dataset.
Furthermore, a new vertex reconstruction algorithm has been developed to precisely reconstruct the position (vertex) of the LLP as well as the resulting b hadrons before their decays, providing important physics information to identify displaced bottom jets. A cutting-edge AI algorithm has also been developed to exploit the physics information of displaced vertices and the associated charged tracks. The algorithm is based on the state-of-the-art graph neural networks (GNNs). A GNN AI algorithm is developed to capture sophisticated correlations between the LLP decay products, and has significantly improved the discrimination between LLP signatures and the overwhelming background processes. Combining these new techniques, this new Run-3 search has achieved remarkable improvements over any other existing search for similar signatures. The observed data are consistent with the background predictions, and currently the most stringent limits are set on the rate of the Higgs boson to decay to LLPs – the limits are ten times better than other existing results despite using a much smaller dataset in comparison! Yet, this is just scratching the surface of the hidden sector and there is still a huge room for discovery.
Figure 2: The upper limits on the rate of the Higgs boson to decay to new long-lived particles, with each of them further decaying into a pair of bottom quarks. The results from previous searches are also shown. Despite using a much smaller dataset, this new search achieves a factor of up to 10 improvement over previous searches.
Looking forward, a much larger dataset is expected to be taken by the end of Run 3, which will significantly expand the coverage of this search. In addition, a new data taking strategy with the parking method enables even better efficiencies for the displaced-jets triggers, allowing even more signal events to be collected. Many more recent LLP triggers have also been developed and implemented in Run 3, enhancing trigger efficiencies across complementary phase spaces. Powered by the new vertex reconstruction and AI techniques, the sensitivity of a wide variety of LLP searches is expected to be significantly improved. This includes searches for heavy neutrinos, axion-like particles, and dark shower signatures, among others. The enhanced CMS discovery potential via LLPs in the ongoing and upcoming LHC runs will provide powerful handles to address long-standing puzzles in particle physics.
Read more about these results:
-
CMS Physics Analysis Summary (EXO-23-013): " Search for low-mass long-lived particles decaying to displaced jets in proton-proton collisions at 13.6 TeV "
-
@CMSExperiment on social media: LinkedIn - facebook - twitter - instagram
- Do you like these briefings and want to get an email notification when there is a new one? Subscribe here