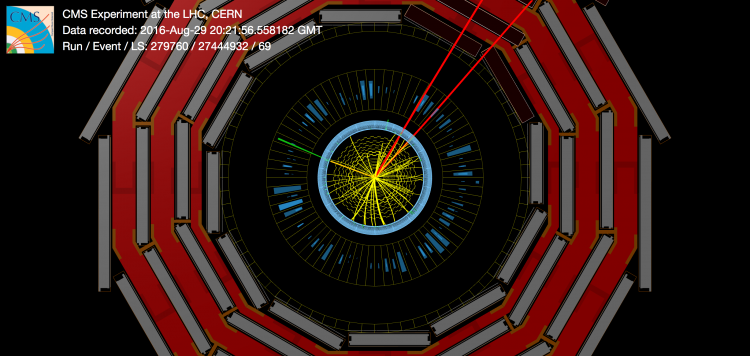
The study of dark matter is one of the primary research topics at the CMS experiment. The standard model of particle physics describes the behavior of elementary particles with incredible accuracy but provides no explanation or possible particle that can constitute dark matter. On the other hand, many astronomical measurements have measured that dark matter accounts for more than 80% of the mass in the observable universe. Besides its existence, physicists know very little about dark matter, due to its seemingly extremely feeble interaction with ordinary matter.
There is a vast number of proposals explaining the identity of dark matter and how to accommodate it in an extension of the standard model. Some of such theoretical models predict that dark matter particles can be produced in the high-energy proton collisions at the large hadron collider, enabling detailed studies of its properties. One class of dark matter models actually introduces a whole new family of particles called the dark sector particles. Most of these new particles would have no direct interaction with the known particles and would therefore collectively act as dark matter, invisible to objects made of "ordinary matter" like humans. Within the dark sector family, however, there whould be new types of particles and interactions.
How would dark sector particles be produced at the large hadron collider? The key could be the Higgs boson. Among the standard model particles, the Higgs boson has a unique combination of properties that could allow it to interact with dark sector particles without introducing other changes to the model. In addition, being the latest particle of the standard model to be discovered, some of the properties of the Higgs boson are still only known within large uncertainties. If there is indeed such an interaction between dark matter and the Higgs boson, and if the mass of the Higgs boson is larger than that of some of those dark sector particles, the former can decay into the latter with some probability.
In particle detectors like the Compact Muon Solenoid, dark sector particles produced in the proton collisions are not directly observed. One of the distinguishing characteristics of the dark sector is that it has very weak or no interaction with the ordinary matter of the detector, meaning the particles just pass through the experiment without leaving a signal. Instead, their production can be inferred from the "missing transverse momentum", the imbalance in the sum of particle momenta in the plane perpendicular to the proton beams, which should add up to zero if all particles are detected.
To test one of the many dark sector models proposed in the literature, CMS physicists set about looking for events where the Higgs boson decays into one photon and one massless "dark photon", which would be the particle equivalent to the photon in electromagnetism, but in the dark sector. Events featuring a photon and missing transverse momentum were analyzed. Because there are processes predicted by the standard model that result in the same final state of a photon and missing transverse momentum, which we call the background processes of this analysis, multiple measures to reject the background while retaining a potential dark photon signal were put in place.
One way to distinguish between dark photons and normal standard model collisions is the use of missing transverse mass (Mt), a quantity that is an estimate of the mass of a particle that decays into a detected and an undetected particle. If the missing transverse momentum indeed arises from a dark photon from the decay of the Higgs boson, the value of Mt will most frequently be close to the mass of the Higgs boson, i.e., at 125 GeV.
A complementary approach is to target only a specific production mode of the Higgs boson, where a Z boson is made in association with the Higgs boson ("ZH production"). A Z boson is a particle that is easily identifiable in the CMS detector when it decays into an electron-positron pair or a muon-antimuon pair. Since there are only few other possible kinds of LHC collisions that produce a photon, missing transverse momentum, and a Z boson, a dark photon signal from this production mode would be unambiguously visible even with a few events.
How many signal events, then, can physicists expect in the billions and billions of LHC collisions? In other words, what is the probability for dark photons in this signature to be generated in proton-proton collisions? The answer is actually "very, very rarely" for realistic values of the physics parameters that agree with the behavior of dark matter in the universe. However, thanks to the enormous data set collected by CMS during Run 2 of the LHC from 2016 to 2018, which corresponds to roughly 10 quadrillion proton-proton collisions, it has become worthwhile to check if there may indeed be a few dark photon events recorded.
The missing transverse mass distribution of the events selected in the analysis. The distribution of the observed events (black points) is statistically consistent with the prediction from the standard model (filled histograms), but not with the presence of dark photon signals at the production rates shown in the figures (green and magenta empty histograms).
The figure is the result of the analysis, showing one of the Mt distributions used in the analysis. The black points correspond to the observed number of events in each Mt bin (for example, the first bin includes values 0 < Mt < 80 GeV) and the colored filled distributions represent the estimated background. The purple and neon green lines illustrate how the data would look if dark photons would be present in the data: events would be distributed slightly differently for two different values of the theoretical model parameters. The observed number of events does not exceed a level that can be explained from background events. Therefore, the results are interpreted as upper bounds on the probability that a signal would have been seen, that can in turn be translated into bounds on which dark matter scenarios are still allowed. That way, this null result will serve to guide further theoretical and experimental work.
An event featuring a muon-antimuon pair (red), a photon (green), and large missing transverse momentum. The invariant mass of the muon-antimuon system is close to the known mass of the Z boson. It is however impossible to tell exactly which process gave rise to the photon and the missing momentum.
Because of the importance of the Higgs boson as a possible "messenger" between the standard model particles and dark matter, this measurement is one of the first of a program of intensive investigation of its various possible decay modes using the Run 2 data. Studies of rare, non-standard decay modes of the Higgs boson are only now becoming realistic with the use of large data sets like the one collected in LHC Run 2 between 2015 and 2018. Indeed, this analysis is one of the first to use the full Run 2 data set. In the coming years, the CMS experiment will be exploring many intriguing theoretical models in collisions that are so rare that they were up to now impossible to observe.
- Log in to post comments