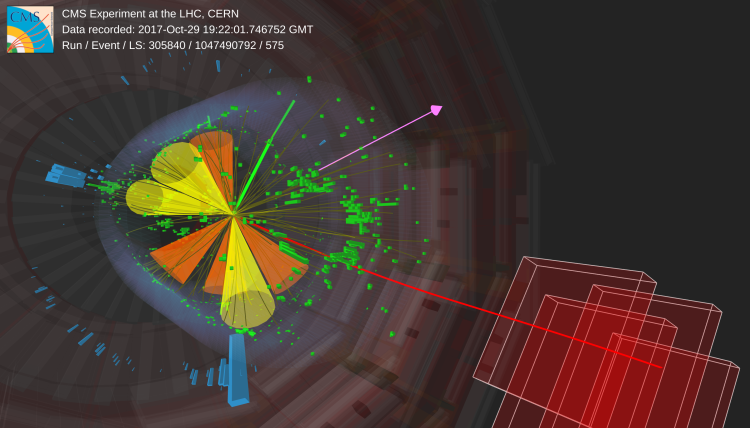
The Higgs boson is deeply connected to the mechanism that generates the masses of elementary particles. In the Standard Model (SM), which describes the properties of all elementary particles and the interactions among them, the Higgs boson interacts with the other particles depending on their mass: the heavier a particle, the stronger the interaction with the Higgs boson. It has the strongest interaction with the top quark, which is the heaviest known elementary particle. Because the coupling between the Higgs boson and the top quark is so strong, it is one of the best places to look for deviations from the SM, which can arise from new physics. In proton-proton collisions at the LHC, a Higgs boson can be produced in association with a top quark-antiquark pair (ttH), which provides a direct probe of the coupling between the top quark and the Higgs boson. In the SM, the Higgs boson can decay in many ways. Among these, the decay to a bottom quark-antiquark pair, which is the second heaviest quark after the top quark, is the most frequent one. The CMS Collaboration has recently published a measurement of the production rate of ttH with the Higgs boson decaying to a bb pair.
The top quark produced in this process decays into a bottom quark and a W boson, which in turn decays either into charged leptons and neutrinos or into quarks. The charged leptons, typically electrons and muons as used in this measurement, can be identified directly in the different sub-detectors of the CMS detector, along with precise measurements of their momentum and energy. On the other hand, the quarks manifest themselves as jets of hadrons. Three different decay channels of the top quark pair are utilized in this measurement therefore ending up with final states containing either zero, one or two charged leptons (electrons/muons). Fig. 1 shows one such candidate ttH event in the CMS detector with an electron and a muon in its final state along with multiple jets which originate from the four bottom quark.
Figure 1: Event display of a candidate ttH event recorded with the CMS detector in 2018, with a final state comprising of an electron (green line), a muon (red line), four jets (orange cones) all identified to originate from bottom quarks, and missing transverse momentum (pink arrow) from particles escaping CMS without being detected, such as neutrinos.
Since the final state of ttH production has a large number of jets originating from bottom quarks, it is crucial to identify them, which is possible due to the much longer lifetime of hadrons from bottom quarks as compared to those from light quarks. The CMS experiment has made significant improvements, using machine learning techniques, to the algorithms to identify jets from bottom quarks and this measurement benefited directly. For ttH production, the final state has four bottom quarks with two coming from the decay of the Higgs boson while the other two from the top quark decays. One of the biggest challenges of this measurement is posed by background processes arising in proton-proton collisions which do not involve a Higgs boson but look very similar and are much more abundant. The dominant background among these comprises of a top quark-antiquark pair produced in association with a bottom quark-antiquark pair (tt+bb), which also has four bottom quarks in the final state, but is several times more likely to occur than ttH. It is therefore critical to have a good understanding of the modeling of this background process in simulation which is used to estimate its production rate. In recent years, significant improvements in the theoretical modeling of this challenging background process has benefited this measurement.
In order to separate the interesting ttH events from the large backgrounds, a group of researchers from several institutes came together to devise sophisticated analysis techniques involving state-of-the-art deep neural networks. The events are first separated based on the total number of jets and number of b-tagged jets in the final state. Neural networks are trained in these separate categories on several kinematic features of simulated signal and background events, and then used to classify the data events as most likely signal or one of the dominant background processes. The observed data is then compared to predictions from simulation in bins of the neural network output in all the separate categories, as shown in Fig. 2, to obtain a measurement of the production rate of ttH.
Figure 2: Observed data (points) from 2018 and expected contributions from signal and background processes (filled histograms) as a function of the neural network score in all categories from the zero (left), one (middle) and two (right) lepton decay channels of the top quark pair.
Figure 3 shows the final result, using data from three years, in terms of the signal strength modifier (μ) which is defined as the ratio of the measured rate of ttH production and the rate predicted by the Standard Model. The ttH production rate is measured to be slightly smaller than the expectation from the SM, although the discrepancy can still result from statistical fluctuations – more data in the coming years will tell for sure!
Figure 3: Measurement of the ttH production rate, separately in each decay channel (top three rows), in each year (middle three rows), and for the combination of all channels and years (lower row).
Written by: Abhisek Datta, for the CMS Collaboration
Edited by: Muhammad Ansar Iqbal
Read more about these results:
-
CMS Publication (HIG-19-011): " Measurement of the ttH and tH production rates in the H→bb decay channel using proton-proton collision data at 13 TeV "
-
Display of collision events: CERN CDS
-
@CMSExperiment on social media: Bluesky - Facebook - Instagram - LinkedIn - TikTok - Twitter/X - YouTube