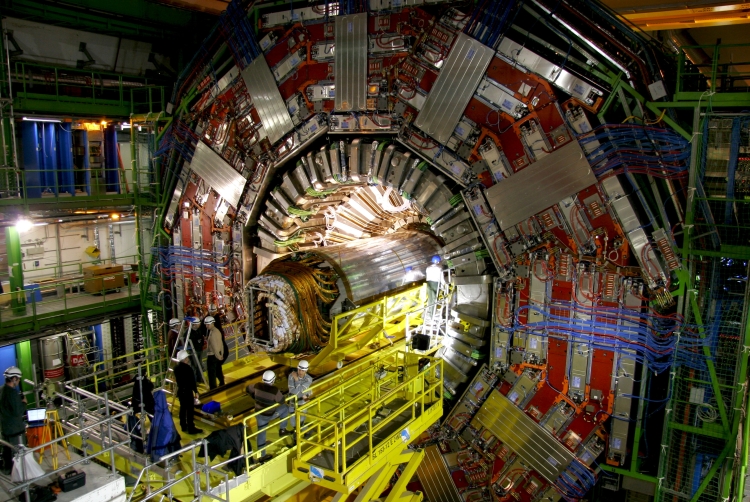
New in! CMS physicists search for heavy cousins of the neutrino in beauty meson decays
Back in 2018, the physicists of the CMS experiment designed and recorded a B-meson-enriched data sample, the “B-Parking” data set. The B mesons (short for beauty mesons) are particles containing a quark and an antiquark, one of them carrying beauty flavor. The B-Parking data set was primarily thought for measurements of production and decay rates of the beauty mesons and searches for flavor anomalies. Little did we know that the B-Parking data set could also play a crucial role in the hunt for Heavy Neutral Leptons (HNLs), the hypothetical heavier and sneakier cousins of the neutrinos.
What are heavy neutrinos and why do we search for them? HNLs are hypothetical heavy particles that only interact with their light counterparts, the neutrinos. They can be a candidate for dark matter, give hints on the possible source of the asymmetry of matter and antimatter in the universe, and explain the smallness of neutrino masses through the see-saw mechanism. These particles can’t be observed directly, as they do not interact with particles we can detect in CMS. However, every once in a while HNLs and light neutrinos can transform into one another. This phenomenon, called “neutrino mixing”, happens with very small probabilities depending on the light neutrino flavors: we denote these probabilities by the symbol |Vℓ|2 for the three lepton flavors (with ℓ = μ for the muon, e for the electron, and τ for the tau lepton). If a transformation happens, we can catch an HNL in disguise! The mixing between light and heavy neutrinos happens with tiny probabilities, hence we need an abundant source of light neutrinos to be able to catch an HNL - and here is where the B mesons come into play!
Historically, ATLAS and CMS physicists have used decays of the W boson as neutrino sources in HNL hunting, as they are quite energetic and therefore easy to disentangle from other processes. At the LHC, B mesons are produced about a thousand times more frequently than W bosons and also decay to neutrinos. On the downside, the decay products of a B meson are less energetic and, therefore, not as easy to identify as those that result from decays of W bosons. The B-Parking data set solves this issue: about 75% of the events collected in this sample host a B meson pair. This has been made possible as the events were selected by requiring the presence of a low-energy muon slightly displaced from the region in which the LHC protons collide. The muon displacement is a blueprint for a B meson, as these particles travel for a few millimeters in the detector before decaying. The B-Parking data set is the ideal “light neutrino haystack” to catch an HNL in disguise!
How do we search for an HNL? A light neutrino mixing with an HNL will carry the properties of the HNL itself: it will live and travel through the detector for more time than what we usually expect for a light neutrino and the combination of its decay products will carry information on the HNL mass. The CMS researchers decided to look for HNL decays to a pion and a lepton: the process appears as a displaced vertex formed by the lepton and the pion.
The vertex displacement is a fundamental feature of this analysis: it greatly helps to reject backgrounds and it is intimately connected to the HNL intrinsic lifetime. Moreover, the B mesons are lighter than the W bosons, and yield decay products with lower energies. The HNL candidates cover less space with respect to heavy neutrinos produced in W boson decays, allowing the researchers to test a broader range of lifetimes. The lepton and the pion are reconstructed with high precision in CMS and, hence, the HNL mass can be determined with virtually perfect accuracy, another unique feature of this analysis! The signal would appear as an excess of events in the spectrum of the invariant mass of the lepton and the pion, as reported in Fig. 1.
Figure 1: An example of the lepton-pion invariant mass spectrum: the green “peak” represents a hypothetical HNL signal with a mass of 2 GeV and a lifetime of 1.7 ns, superimposed on the data distribution (black dots); the background prediction is also shown (red curve).
No evidence of an excess over the background-only prediction has been found in this search. CMS physicists have therefore turned the results into exclusion bounds on the neutrino mixing amplitudes |Vℓ|2. These are linked to the probability for the HNL to mix with a light neutrino of a specific flavor. In the results the |Vℓ|2 are parametrised as (re,rμ,rτ) where for every lepton flavor ℓ, rℓ = |Vℓ|2/|VN|2 and the denominator is defined as |Ve|2+|Vμ|2+|Vτ|2. Various (re,rμ,rτ) combinations as a function of the HNL mass have been constrained: the limits obtained under the hypothesis that the HNL mixes only with muon neutrino are reported in Fig. 2 as an example. Moreover, exclusion bounds on the HNL cτ (where τ is the HNL lifetime) for any possible flavor coupling configuration (re,rμ,rτ) have been computed for the first time for masses 1 and 1.5 GeV (Fig. 3). In the landscape of HNL searches, this novel approach of an HNL search in B meson decays sets the most stringent constraints among those obtained at collider experiments, for HNL masses between 1 and 2 GeV, and complements the CMS multifaceted approach to heavy neutrino physics.
Figure 2. Expected and observed exclusion limits (at 95% confidence level) on the probability amplitude for an HNL to mix with a muon, here labeled |VN|2 , as a function of mN, in the Majorana scenario.
|
|
Figure 3: Observed lower limits (at 95% confidence level) on cτN as functions of the mixing ratios (re,rμ,rτ) for mN = 1.0 GeV (left) and 1.5 GeV (right) in the Dirac scenario.
Read more about these results:
-
CMS Paper (EXO-22-019): " Search for long-lived heavy neutrinos in the decays of B mesons produced in proton-proton collisions at 13 TeV "
-
@CMSExperiment on social media: LinkedIn - facebook - twitter - instagram
- Do you like these briefings and want to get an email notification when there is a new one? Subscribe here