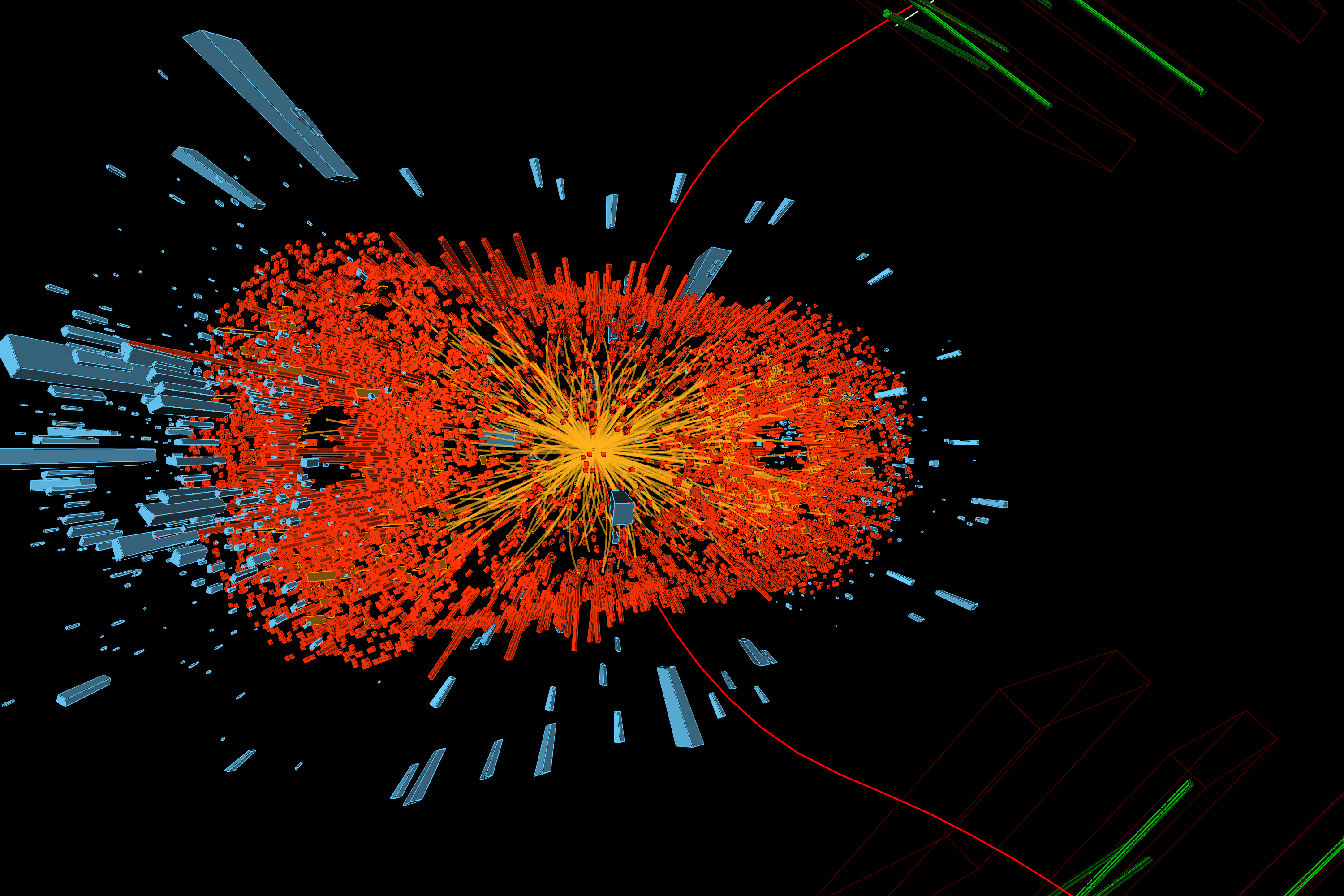
In 2011, CMS presented early evidence that Upsilon (Υ) particles produced in lead-lead collisions “melt” as a consequence of interacting with the hot nuclear matter created in these heavy-ion interactions. CMS has since updated and extended this result using additional data collected in the 2011 heavy-ion run, and the observation now has a significance of greater than 5σ (or 5 standard deviations), the gold standard for claiming a discovery in high-energy physics.
Hot nuclear matter
The familiar proton is a composite particle made up of quarks held together by gluons. One of the properties of these elementary quarks and gluons is that they cannot exist freely and are always bound together to form hadrons. At least not at the temperatures we come across daily: Our best understanding of the early Universe tells us that at the very high temperatures prevalent in the first microseconds following the Big Bang, quarks and gluons existed freely as a homogenous medium called the quark-gluon plasma (QGP).
Smashing heavy nuclei inside particle colliders creates a hot nuclear matter thought to be similar to the primordial QGP. Apart from colliding protons, the LHC dedicates beam-time to colliding lead nuclei (Pb) and the resulting collisions have provided physicists with a wealth of valuable data to study the hot nuclear matter. While it isn’t possible to observe this medium directly, its presence can be inferred based on the expected behaviour of the particles that interact with it.
A literal melting pot
To explore the properties of the medium, CMS compares the properties of the particles produced in heavy-ion collisions (PbPb) with those produced in proton collisions (pp). A novel candidate for these analyses is the Υ particle, a meson made of a bottom quark and its own antiparticle. Just as an atom can exist in an excited state — an electron can receive some energy and move to a higher orbital — so can composite particles such as the Υ. The Υ(1S) state is the ground state, while excited states include the Υ(2S) and Υ(3S) that are more loosely bound than the ground state. A remarkable aspect of this measurement is that CMS is the first experiment to successfully separate the signals of the three Υ states in heavy-ion collisions, thanks to the superb mass resolution of the CMS detector.
Theorists predicted in the mid-’80s that having to traverse the hot medium will cause particles such as Υs, produced in these interactions, to “melt” or be suppressed in it. It is important to note that this suppression doesn’t mean that these particles weren’t produced in the first place, but that the bound states were possibly destroyed as a result of interacting with the medium before they could decay into muons. The more loosely bound the state, the more easily it would melt; so, the (3S) state would be suppressed more than the (2S) state, while the (1S) state would be the least suppressed of the three.
Observing such a sequential suppression in the number of Υ particles detected in collisions of lead nuclei relative to those in proton collisions at the same energies helps probe the properties of the hot, dense medium.
The previous CMS analysis used just 7.3 μb−1 (inverse microbarns) of PbPb data from 2010, whereas the new study uses 20 times as much data: 150 μb−1 of PbPb collected in 2011. Since the lead-ion runs took place at a per-nucleon collision energy of 2.76 TeV and the proton run at the time was at 7 TeV, the LHC provided a special pp run in early 2011 at 2.76 TeV, with CMS collecting around 230 nb−1 (inverse nanobarns) of data. CMS measured the decays of the three Υ states into two muons in both PbPb and pp collisions and found that the rates in the former were significantly suppressed compared to the latter, as expected if a QGP were formed. The ratio of Υ(2S) to Υ(1S) in PbPb collisions was found to be approximately 21% that of the same ratio in pp collisions. Similarly, the ratio of Υ(3S) to Υ(1S) was found to be only around 6% in PbPb collisions relative to those with protons.
In addition, all three states were found to be suppressed in PbPb collisions. The suppression is further demonstrated by the fact that it is more pronounced in very central heavy-ion collisions — ones where the nuclei collide head-on (higher Npart in Figure 2) — as opposed to peripheral collisions. This behaviour is expected as central collisions release more energy and the hot nuclear matter would consequently have a higher temperature, thereby melting more particles. And because the more excited states melt at lower temperatures, the sequential suppression is an excellent indicator of the temperature of the nuclear medium produced in PbPb collisions.
The 2011 results had a significance of 2.4σ; the current results are over 5σ in significance, implying a probability of less than one in 3.5 million of obtaining a false positive. This observation represents an important milestone in the study of heavy-ion collisions and has been made possible by the excellent performance of the LHC itself as well as collaborative efforts between the particle-physics (pp) and nuclear-physics (PbPb) groups at CMS. In early 2013, the LHC will smash protons with lead nuclei, and the resulting cold nuclear matter will help analyse the intricacies of the observed sequential suppression.
Other resources
- Log in to post comments