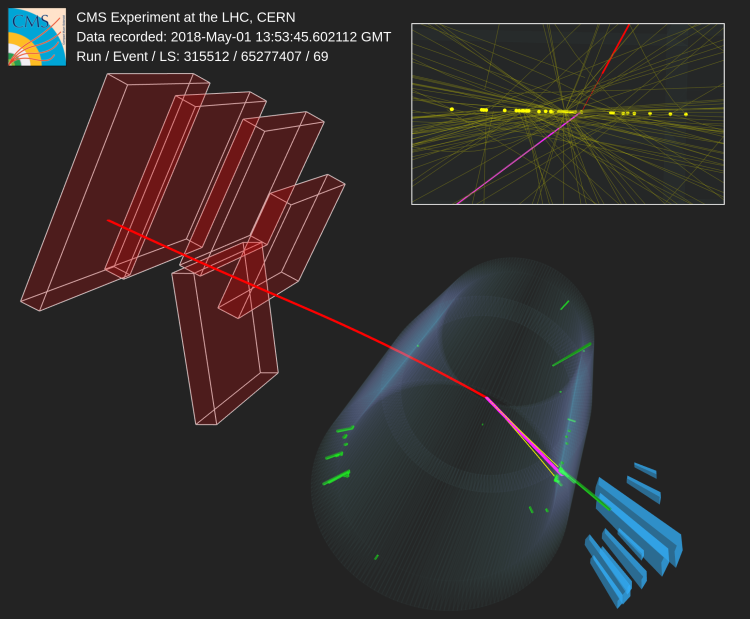
In quantum electrodynamics (QED), the quantum theory of electromagnetic interactions, the magnetic dipole moment of a particle acquires quantum corrections originating from interactions with virtual photons and other particles, often referred to as the anomalous magnetic moment of that particle. The first order correction was calculated already in 1948 by Julian Schwinger, deriving the renowned value of α/2π. Schwinger later shared the Nobel Prize in Physics with Sin-Itiro Tomonaga and Richard Feynman "for their fundamental work in quantum electrodynamics, with deep-ploughing consequences for the physics of elementary particles". The anomalous magnetic moment of the electron stands as one of the most precise measured quantities in physics. It manifests the principles of QED and aligns remarkably well with theoretical predictions, achieving an extraordinary precision of up to ten decimal digits. The muon's anomalous magnetic moment has also been measured to a very high degree of accuracy. Yet, unlike the case with the electron, there is a persistent discrepancy between the experimental measurements and theoretical predictions, indicating either that some detail in the calculations is not accounted for or the presence of physics beyond the Standard Model (SM). The determination of the magnetic moment of the heaviest SM lepton, the tau lepton, suffers from a lack of high-precision experimental data, since its short lifetime makes direct measurements exceedingly challenging. Martin Perl, known for his discovery of the tau lepton, described in one of his talks after receiving the Nobel Prize in Physics that the precise measurement of the tau magnetic moment with enough precision to validate the Schwinger term is still a dream. At the time, the uncertainty achieved in measurements was approximately 30 times larger than the precision needed for this validation.
Experimental insights into the tau anomalous magnetic moment can be indirectly obtained by measuring the exclusive production of tau lepton pairs from two-photon collisions, where the magnitude of the anomalous magnetic moment impacts the production rates. As two charged particles pass each other at relativistic velocities they generate intense electromagnetic fields, leading to photon collisions (Fig. 1).
Figure 1. Production of τ lepton pairs by γγ fusion in three topologies: exclusive (left), single proton dissociation or semiexclusive (middle), and double proton dissociation (right).
The first significant studies were performed at the Large Electron–Positron Collider (LEP), which finally managed to reduce the experimental uncertainty to "only" 20 times larger than the desired precision, holding the world's best measurement since 2004. At the LHC, the ATLAS and CMS collaborations tried to improve the precision using ultra-peripheral heavy ion collisions, but could not surpass the two-decade-old result.
Photon collisions differ substantially from proton collisions because the proton can emerge intact and most of the collision energy is focused on the photon-photon interaction. As a result, final state particles are produced exclusively, with no other particles coming from the same vertex. Such a signature was easily observed during the first collisions at the LHC, but detecting such events with increasing instantaneous luminosities and higher pileup rates during the LHC Run 2 became increasingly challenging. One way to study photon-induced processes is to tag intact protons, as was demonstrated by the CMS collaboration more than a year ago. The other way, thought to be unrealistically challenging, is to search for an exclusive signature, now successfully demonstrated by the CMS collaboration with the first observation of photon-induced production of tau lepton pairs in proton-proton collisions at the LHC.
Figure 2: Candidate γγ → ττ event measured in proton-proton collisions by CMS. The event is reconstructed as having a leptonic τ decay, τ → μνν, with the μ track indicated in red, and a hadronic τ decay, τ→πππν, with the 3 charged pions indicated by the yellow tracks and by the energy deposits in the ECAL (green) and HCAL (cyan). Find this 3-dimensional interactive version with all tracks here.
The exclusive production processes in heavy ion collisions are identified thanks to the unique signature of an almost empty detector, while the main drawback is that the photons are produced with lower energies. Since the sensitivity to the quantum corrections increases with the collision energy, photon-induced processes in high-energy proton-proton collision should be a better way to probe the tau lepton anomalous magnetic moment. The supreme tracking capabilities of the CMS detectors allow the isolation of interaction vertices from pileup collisions and resolving the exclusive signature; the header figure shows an event display before (inset) and after cleaning the pileup tracks. However, the accurate modeling of inclusive backgrounds featuring a small number of reconstructed tracks always posed a big challenge. It was now successfully surmounted, for the first time, through the use of sophisticated analysis techniques, so that we could finally observe the exclusive production of tau lepton pairs in proton-proton collisions. “This remarkable achievement of detecting ultra-peripheral proton-proton collisions sets the stage for many groundbreaking measurements of this kind with the CMS experiment” said Michael Pitt, from the CMS analysis team.
Analyzing the energy spectra of the particles emitted in the decay of the two tau leptons provides sensitive information on the quantum corrections, by probing the contribution of electromagnetic moments to the photon-tau vertex. Figure 3 shows the results, in terms of the anomalous magnetic dipole moment (aτ).
Figure 3. Measured values of the anomalous magnetic moment (aτ), compared with previous results.
The results significantly improve our constraints on the anomalous magnetic moment of tau leptons, with an uncertainty that is only three times larger than the Schwinger correction. “It is truly exciting that we can finally narrow down some of the basic properties of the elusive tau lepton”, said Izaak Neutelings, another member of the analysis team. "This analysis introduces a novel approach to probe tau g-2 and revitalizes measurements that have remained stagnant for more than two decades", added Xuelong Qin. This remarkable achievement paves the way for a new pursuit towards the coveted precision, which should eventually turn the long-held dream into reality.
Read more about these results:
-
CMS Physics Analysis Summary (SMP-23-005): "Observation of γγ → ττ in proton-proton collisions and limits on the anomalous electromagnetic moments of the τ lepton "
-
Display of collision events: CERN CDS
-
@CMSExperiment on social media: LinkedIn - facebook - twitter - instagram
- Do you like these briefings and want to get an email notification when there is a new one? Subscribe here