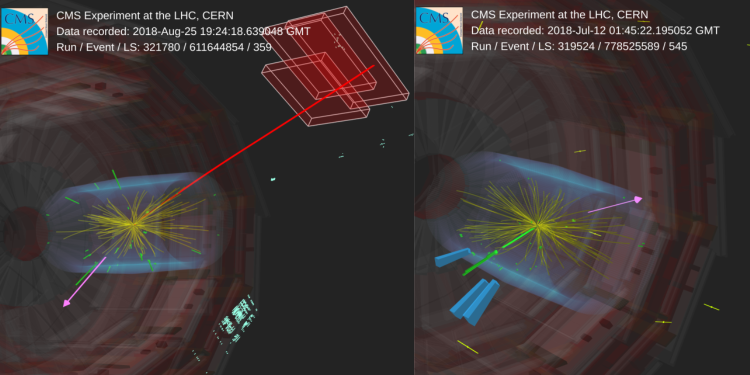
Two key properties of neutrinos in the standard model of particle physics (SM) are that they are massless and “left-handed”, i.e. their spin is always opposite to their momentum. The observation of neutrino oscillations posed a big challenge to the completeness of the SM, by implying that neutrinos are massive. Furthermore, being at least a million times lighter than the electron (the lightest massive fermion in the SM) may suggest that the neutrinos get their mass generated by a different mechanism. The puzzle of the neutrino mass can be solved by introducing a “right-handed” partner to the neutrino, often called “heavy neutral lepton” (HNL). With a “right-handed” partner, the SM neutrinos can have masses, just like the other SM particles. These heavier cousins of the SM neutrinos are not supposed to directly interact with the SM particles through the electroweak or strong interactions, but can be produced through mixing, together with the SM electron, muon, and tau neutrinos. Besides solving the neutrino mass problem, models with HNLs can also explain other SM mysteries, such as the matter-antimatter asymmetry of the universe through CP violation, the nature of dark matter, and the anomalous magnetic moment of the muon.
Experiments have been searching for HNLs for decades, but it is challenging: we do not know their mass nor the strength of their interaction with the SM leptons. How they could be seen in an experiment strongly depends on these parameters. In particular, if their mass is small enough and/or their mixing with SM leptons is weak enough, HNLs could travel macroscopic distances (up to several meters) before decaying. Such “long-lived” particles (LLPs) are particularly difficult to detect in the CMS experiment, which was not designed for that purpose. Nevertheless, CMS physicists recently established that LLPs could be seen in the muon detectors. When the long-lived HNL decays, it produces a cascade of particles (a shower) that is measured by the gas detectors as a concentrated outburst of signals, unlike anything that happens for muons or other SM particles produced in the LHC collisions. Similarly to what happens for jets, the larger is the energy of the LLP, the larger will be the particle shower, activating a larger number of channels in the detectors. This signature is commonly referred to as Muon Detector Shower (MDS).
Figure 1: Illustration of the production and decay of a heavy neutral lepton (N).
The CMS physicists searched for these HNL showers in events with a W-boson decay, because the W boson usually decays into an energetic electron or muon plus a SM neutrino, which can turn into an HNL (as illustrated in Fig. 1). The energetic electrons or muons lead to very clean signatures in the detectors, allowing us to trigger (select) these events and collect them for offline analysis, where they are further selected by imposing that the MDS is seen in the hemisphere opposite to that of the electron or muon.
Not many other processes can mimic the same configuration (a lepton and an MDS). A very energetic jet can “punch-through” the hadron calorimeter and deposit some of its energy in the muon system, creating an MDS. A high-energy muon can sometimes radiate energy while passing through the steel placed between the muon detectors, leaving more hits than usual, like an MDS. Most of these background processes can be suppressed by requiring that there are no jets or muons reconstructed in the direction of the MDS. The analysis also rejects events with the MDS located in parts of the detector more susceptible to background. Applying these requirements reduces the level of background by a factor of around one million. A distinctive MDS feature is the number of hits in the clusters (Nhits): signal events tend to have bigger clusters than background events. A threshold in Nhits has been determined, using simulated signal events, to retain a large fraction of the HNL signal events while rejecting most of the remaining background. Using a control region in data where no signal is expected, one can predict how many events are expected in the search region: the observation closely matches the prediction. Figure 2 shows two events with some of the largest clusters in the search region.
![]() |
![]() |
Figure 2: Two HNL candidate events with an MDS in the end-cap (left, in light blue color) and in the barrel (right, in bright green color) plus a back-to-back muon (left) or electron (right). The electron/muon are back-to-back with the MDS. The clusters have 243 and 203 hits, respectively for the muon and electron events.
Given the absence of HNL signals in the data, the ranges of HNL masses and couplings that would have produced a clear signature in the data have been excluded, as can be seen in Fig. 3. The excluded parameters region correspond to a HNL that could typically travel for meters before decaying, for which MDS is particularly effective to capture.
The MDS signature is being improved for further searches for new physics. In Run 3 the ability to trigger on the MDS object has been added, so that CMS will be able to collect much more efficiently events with potential LLP decays in the muon system, increasing the ability to detect new physics signals by at least an order of magnitude.
![]() |
![]() |
Figure 3: Exclusion limits on the coupling of a Dirac HNL to the muon-neutrino (left) and electron-neutrino (right) as a function of the HNL mass. Results from other analyses are shown in shaded bands. The new analysis sets the best limit around 2-3 GeV
Read more about these results:
-
CMS Physics Analysis Summary "Search for long-lived heavy neutral leptons decaying in the CMS muon detectors in proton-proton collisions at 13 TeV"
-
Display of collision events: CERN CDS
-
@CMSExperiment on social media: LinkedIn - facebook - twitter - instagram
- Do you like these briefings and want to get an email notification when there is a new one? Subscribe here