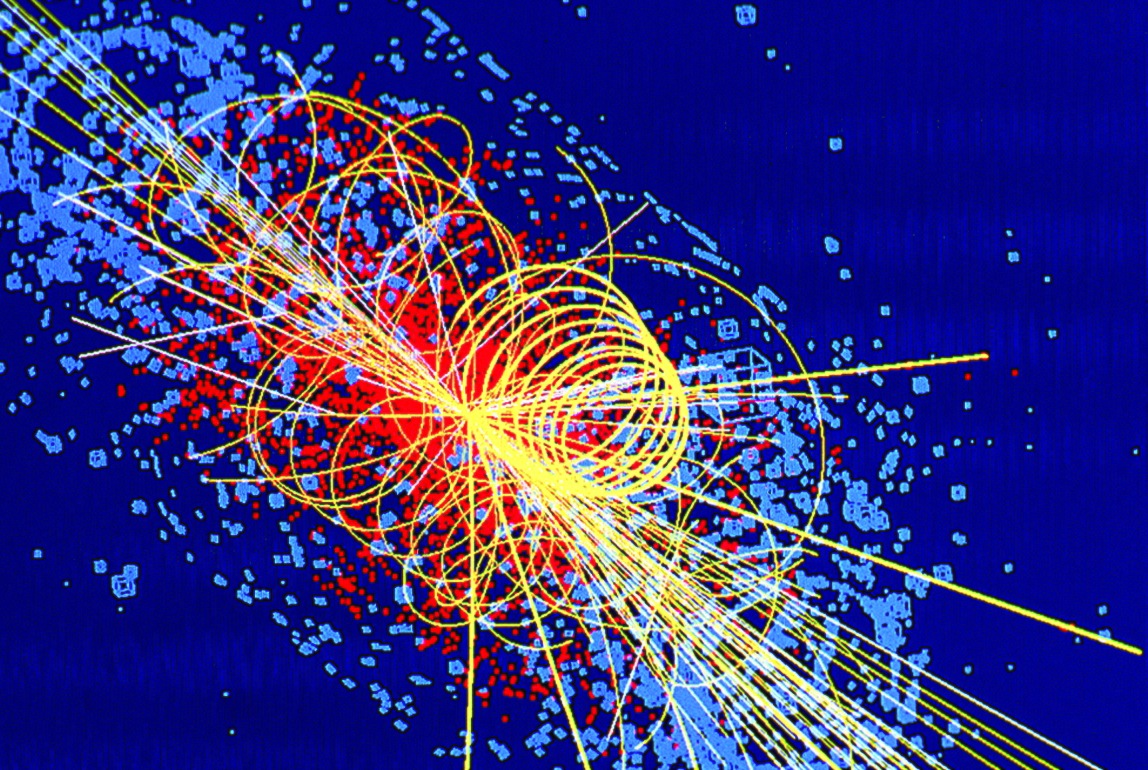
The discovery of the Higgs boson, one of the main goals of the CMS experiment, proved that the Higgs field exists. When a Higgs boson is formed in an LHC collision, it decays very quickly to other particles. Therefore, we have to study these decay products, the 'signature' the Higgs boson leaves behind, to learn about the Higgs boson. When the Higgs boson was first observed, it was seen in decays to pairs of Z-bosons, with subsequent decay into four leptons (particles such as electrons or muons), as well as in decays to pairs of photons.
The event shows characteristics expected from the decay of the SM Higgs boson to a pair of Z bosons, one of which subsequently decays to a pair of electrons (green lines and green towers) and the other Z decays to a pair of muons (red lines).
Photons are detected in the electromagnetic calorimeter. In the first image we see the detector head-on with the electromagnetic calorimeter (ECAL) in green. Green trajectories show these photons, while the blue trajectories show other particles emerging from the collision with the red marks showing hits in the hadron calorimeter. The ECAL is able to tell the mass of the particle to better than 1%.
Event recorded with the CMS detector in 2012 at a proton-proton centre of mass energy of 8 TeV. The event shows characteristics expected from the decay of the SM Higgs boson to a pair of photons (dashed yellow lines and green towers).
You can watch this video series to learn more about how the Higgs boson was discovered. https://www.youtube-nocookie.com/watch?v=so2nCu2Jkbc
Now that the Higgs boson has been discovered, at CMS, we're making further measurements of its properties. We're not just looking at the two discovery channels for this, but also at other major Higgs boson decay modes: into pairs of b-quarks, tau leptons, and W-bosons... If you'd like to know more, this video series sheds some more light on these different channels.
- Printer-friendly version
- Log in to post comments
- Blinding and unblinding analyses
- Should you get excited by your data? Let the Look-Elsewhere Effect decide